A Compendium of Geochemistry: From Solar Nebula to the Human Brain available in Hardcover, eBook
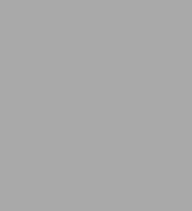
A Compendium of Geochemistry: From Solar Nebula to the Human Brain
- ISBN-10:
- 0691009384
- ISBN-13:
- 9780691009384
- Pub. Date:
- 10/22/2000
- Publisher:
- Princeton University Press
- ISBN-10:
- 0691009384
- ISBN-13:
- 9780691009384
- Pub. Date:
- 10/22/2000
- Publisher:
- Princeton University Press
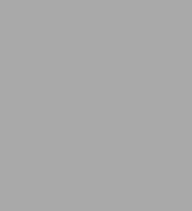
A Compendium of Geochemistry: From Solar Nebula to the Human Brain
Buy New
$91.00Buy Used
$42.02-
-
SHIP THIS ITEM
Temporarily Out of Stock Online
Please check back later for updated availability.
-
Overview
The book focuses on compositional data and related references for such substances as solar photosphere, meteorites, igneous rocks, soils, sedimentary rocks, surficial waters, marine and terrestrial organisms (including humans), and aerosols. It emphasizes the use of original raw data as much as possible, and applies the statistical technique of factor analysis to elucidate any underlying interrelationships among chemical elements and given sample sets. Whenever applicable, simple chemical thermodynamic models are introduced to explain the observed partitioning of elements among different phases.
Product Details
ISBN-13: | 9780691009384 |
---|---|
Publisher: | Princeton University Press |
Publication date: | 10/22/2000 |
Edition description: | New Edition |
Pages: | 440 |
Product dimensions: | 6.00(w) x 9.25(h) x (d) |
About the Author
Read an Excerpt
A Compendium of Geochemistry
From Solar Nebula to the Human BrainBy Yuan-Hui Li
Princeton University Press
Copyright © 2004 Princeton University PressAll right reserved.
ISBN: 978-0-691-00938-4
Chapter One
ATOMS, NUCLEI, AND ENERGY
Introduction
In principle, the distribution of the elements among coexisting phases in equilibrium can be predicted, if one knows the Gibbs free energies of formation and activity coefficients of all chemical species in all phases as functions of temperature, pressure, and compositions of phases. Because this is not always possible, many geochemists attempt to explain and predict the geochemical behavior of various elements by their atomic properties, such as electron configuration, ionization energies, ionic radii, ionic potentials, electric polarizability, crystal lattice energies, hydrolysis constants, solubility products, and electronegativity. This kind of approach has proved to be useful but has also caused confusion when the exact physical meaning of those parameters is ignored.
In order to provide a framework for the discussion in the following chapters, these atomic properties and their closely interrelated nature are reviewed in the following sections. For ease of explaining the observed relative abundance of nuclides in the solar nebula in the next chapter, some pertinent nuclear properties are also reviewed here.
I-1. Periodic Table ofthe Elements and Electron Configurations of Atoms
An atom consists of a nucleus containing protons and neutrons, with electrons orbiting around the nucleus. In an electrically neutral atom, the number of electrons is equal to the number of protons. The number of protons in the nucleus of an atom is called the atomic number (Z). There are 112 known chemical elements so far, as shown in the periodic table (Table I-1a). Elements with atomic numbers between 104 and 112 are artificial radionuclides with short half-lives. The names and symbols for these elements are still contested (Table I-1b). The periodic table consists of seven horizontal rows or periods. The vertical columns under the arabic numerals with A and B are called groups of chemical elements. Elements in the same group are called congeners and have similar physicochemical properties. Therefore, the periodic law states that the physicochemical properties of the elements change in periodic or repeated cycles as their atomic numbers increase. For example, the molar atomic volume of the elements (=atomic weight/density) at 25°C and 1 atmosphere changes periodically as a function of the atomic number (Figure I-1). For some elements that are in the gaseous state at 25°C and 1 atmosphere pressure, the density in the liquid state at the melting point is used.
The similarity in physicochemical properties among certain elements is often a reflection of their similar electron configurations. According to quantum mechanics, the energy levels (or states) and most probable spatial distribution of electrons in the orbital cloud around an atomic nucleus can be described by four quantum numbers, i.e., n (principal), l (angular momentum), [m.sub.l] (magnetic), and [m.sub.s] (spin). For the physical meaning of the four quantum numbers, one should refer to any standard physical chemistry textbook. According to the Pauli exclusion principle, no two electrons in an atom can have the same four quantum numbers.
The principal quantum number, n, is a positive integer that ranges from 1 to 7 for the known atoms in the ground (i.e., lowest-energy) state. The principal energy levels or states with n = 1 to 7 are often denoted as the K, L, M, N, O, P, and Q shells, respectively. For a given n, the angular momentum quantum numbers, l, are positive integers from 0 to n - 1. For example, if n = 4 then l = 0, 1, 2, 3. The energy levels with l = 0, 1, 2, 3, 4 are designated as the s, p, d, f, and g subshells, respectively.
The combinations of n and l can define all the major subshells in atoms. For example, nl = 1s; 2s, 2p; 3s, 3p, 3d; 4s, 4p, 4d, 4f; 5s, 5p, 5d, 5f, 5g, etc. The relative energy levels of these subshells for neutral atoms in the ground state are schematically shown in Figure I-2 (from the lowest 1s to the highest 6d; notice that the g subshell is unnecessary for accommodating electrons of atoms in the ground state). One should be aware that the relative energy levels of subshells are slightly different from those of Figure I-2 when the atoms are positively charged (i.e., cations). This point will be discussed later.
For a given l value, the magnetic quantum number, [m.sub.l], can have all integers between -l and l including 0 (e.g., l = 3, [m.sub.l] = -3,-2,-1, 0, 1, 2, 3). Thus, there are (2l+1) values of [m.sub.l] for a given l. In addition, the spin quantum number, [m.sub.s], can have only two values (i.e., +1/2 and -1/2). Therefore, the combination of [m.sub.l] and [m.sub.s] produces 2(2l + 1) numbers of distinctive quantum states of electrons with the same energy level, i.e., 2(2l + 1)-fold energy degeneracy. In other words, the s subshell (l = 0) consists of one orbital; the p subshell (l = 1) has 3 orbitals; the d subshell (l = 2) has 5 orbitals; and the f subshell (l = 3) has 7 orbitals with the same energy levels. Each orbital can accommodate one pair of electrons spinning in opposite directions, schematically shown in Figure I-2 as cubic boxes with up and down triangles. The electron configuration of any atom with atomic number Z is constructed by feeding electrons one by one into the lowest empty orbitals of subshells until a number of electrons equal to the atomic number Z are all accommodated. The orbitals in the p, d, and f subshells should each be first filled by a single electron with parallel spin before electrons with opposite spins can enter any of those orbitals. For example, the electron configuration of Mn (Z = 25) is [Ar][3d.sup.5]4[s.sup.2], where the superscripts represent the numbers of electrons in subshells and [Ar] the electron configuration equivalent to the Ar atom. [3d.sup.5] represents 5 electrons in the 3d subshell, and these 5 electrons are spinning in the same direction (parallel spins), each in one orbital. There are, however, many exceptions in Figure I-2: the energy levels of (n + 1)s and (n - 1)f are usually slightly lower than those of nd for most of the elements in Figure I-2, but the reverse is the case for some atoms (indicated by solid triangles). In this case, one or two electrons from the (n + 1)s and (n - 1)f subshells should move into the nd subshell. For example, the electron configurations of Cu (Z = 29) and Ce (Z = 58) are [Ar]3[d.sup.9]4[s.sup.2] and [Xe]4[f.sup.2]6[s.sup.2] according to Figure I-2 but in fact should be [Ar]3[d.sup.10]4[s.sup.1] and [Xe]4[f.sup.1]5[d.sup.1]6[s.sup.2], respectively.
The outermost two or three subshells are usually called the subshells of valence electrons. By convention, the subshells of the valence electrons are listed in increasing order of the principal quantum number as shown in Table I-1b (not in order of the subshell energy levels for the neutral atoms as shown in Figure I-2). The advantage of this convention is that the sequence of subshells of valence electrons is listed exactly in the increasing order of energy levels for cations. Therefore, one can easily obtain the electron configuration for any cation, [M.sup.+z], by simply taking away z electrons from the outermost subshell(s) of the neutral atom as given in Table I-1b.
The heavy zigzag line in Table I-1a separates the elements into metals on the left and nonmetals on the right. The elements bordering the zigzag line, such as B, Si, Ge, As, Sb, Te, and Po, are called metalloids. The characteristic properties of all metals are high electric and thermal conductivity, high reflectivity (metallic luster), mechanical ductility, and malleability, and their power to replace hydrogen in acids. The elements can also be classified into four blocks according to which subshell is filled by the last valence electron. They are the s-block of the alkali (group 1A) and alkaline earth metals (group 2A), the d-block of the transition metals (group 1B to 8B), the f-block of the lanthanides and actinides, and the p-block of group 3A to 8A as shown in Table I-1a and Figure I-2.
I-2. Atomic Nuclei and Nuclear Binding Energies
The total number of protons Z and neutrons N in an atomic nucleus is called the mass number A(= Z + N) of the nucleus. Nuclei with identical Z but different N are called isotopes, whereas nuclei with identical N but different Z are called isotones. Nuclei with identical A but variable N and Z are isobars. By convention, an atomic nucleus is represented by a chemical symbol with its mass number A as a left-hand side superscript and its atomic number Z as a left-hand side subscript, e.g., [sup.16.sub.8]O and [sup.12.sub.6]C. Since the elemental symbol already implies the atomic number, the subscript of the atomic number is often omitted, e.g., [sup.16]O and [sup.12]C.
There are about 268 stable nuclei in nature: 159 nuclei with even Z and even N; 55 with even Z and odd N; 49 with odd Z and even N; and only 5 with odd Z and odd N combinations (i.e., [sup.2.sub.1]H, [sup.6.sub.3]Li, [sup.10.sub.5]B, [sup.14.sub.7]N, and [sup.50.sub.23]V). It is apparent that nuclei with paired protons and/or paired neutrons are favored among stable nuclei. Figure I-3 also shows that nuclei with even Z (or even N) usually have more than three stable isotopes (or isotones), and nuclei with odd Z (or odd N) usually have only one isotope (or isotone), or occasionally two. Nuclei with odd A (i.e., even Z-odd N or odd Z-even N) have only one stable isobar, while nuclei with even A (i.e., even Z-even N, except for the five odd Z-odd N nuclei mentioned earlier) have one to three stable isobars. The neutron to proton ratio (N/Z) is about one for stable nuclei with Z (or N) less than 20, but increases gradually with increasing Z and reaches about 1.5 for the heaviest stable nucleus [sup.209.sub.83]Bi (Figure I-3). Any nucleus with Z and N values falling outside the stable nuclei field in Figure I-3 is unstable, and will undergo various radioactive decay steps (such as [alpha] and s decays and spontaneous fission) so that the N/Z ratio will eventually approach one of those stable nuclei.
According to international convention, the mass of one mole of [sup.12]C atoms (= 6.022045 × [10.sup.23] atoms = Avogadro's number) equals 12 grams, and the mass of one [sup.12]C atom is defined to equal 12 atomic mass units (amu). Therefore one atomic mass unit (amu) is equivalent to 1/(6.022045 × 10[2.sup.3]) = 1.66056 × [10.sup.-24] g. Its energy equivalent is 1.492442 × [10.sup.-3] erg = 931.5023 MeV (1 MeV = [10.sup.6] electronvolts). Accordingly, the rest mass of one hydrogen atom [M.sub.H] (one proton plus one electron) is 1.007825037 amu = 938.79134 MeV; the rest mass of aneutron [M.sub.n] = 1.008665012 amu = 939.57378 MeV; and the rest mass of an electron [M.sub.e] = 0.00054858026 amu = 0.5110034 MeV. The masses of various neutral atoms are summarized by Bievre et al. (1984).
The energy released in the formation of a nucleus from its component nucleons (i.e., hydrogen atoms and neutrons) is called the binding energy of the nucleus, [Q.sub.b], i.e.,
[MATHEMATICAL EXPRESSION NOT REPRODUCIBLE IN ASCII]. (I-1)
For example, the binding energy of the [sup.4.sub.2]He nucleus is
[MATHEMATICAL EXPRESSION NOT REPRODUCIBLE IN ASCII].
The relative stability of nuclei can be represented by the binding energy per nucleon ([Q.sub.b]/A). Figure I-4 shows the [Q.sub.b]/A values calculated from equation I-1 as a function of A for all stable nuclei and for some heavy radioactive nuclei (A 226). The complete listing of [Q.sub.b]/A values is given by Yoshihara et al. (1985). The [Q.sub.b]/A values generally increase with increasing A up to a broad maximum around A = 56, which corresponds to the [sup.56]Fe nucleus, then decrease gradually. Therefore [sup.56]Fe is the most stable of all nuclei. Energetically it is possible to release nuclear energies (exothermic process) by fusing more than two lighter nuclei (A < 56) to form a new nucleus with a higher [Q.sub.b]/A value (fusion process). For example,
[MATHEMATICAL EXPRESSION NOT REPRODUCIBLE IN ASCII]. (I-2a)
For nuclei with A > 56, the only way to release the nuclear energy is to split anucleus into lighter nuclei with higher [Q.sub.b]/A values (fission process). For example,
[sup.235.sub.92]U + n [right arrow] [sup.140.sub.56]Ba + [sup.93.sub.36]Kr + 3n + Q; Q = 174 MeV. (I-2b)
In Figure I-4, the [Q.sub.b]/A values for [sup.4.sub.2]He, [sup.12.sub.6]C, [sup.16.sub.8]O, [sup.20.sub.10]Ne, and [sup.24.sub.12]Mg are higher than those of their immediate neighbors. In other words, the nuclei that are multiples of the helium nucleus have extra stability. This extra stability can be seen clearly in the theoretical minimum energy required to remove one neutron,
[MATHEMATICAL EXPRESSION NOT REPRODUCIBLE IN ASCII], (I-2c)
or one proton,
[MATHEMATICAL EXPRESSION NOT REPRODUCIBLE IN ASCII], (I-2d)
from a nucleus as shown in Figure I-5 for [Q.sub.n].
Analogous to the ions with noble gas electron configuration are the nuclei with neutron or proton numbers of 2, 8, 20, 28, 50, 82, and 126, which also have extra stability. Those numbers are called magic numbers. Nuclei with N and/or Z = 8, 50, 82, and 126 also have very low capture cross sections for thermal neutrons (Friedlander et al., 1981).
For a set of isobars, the term (Z x [M.sub.H] + N x [M.sub.n]) in equation I-1 is near constant; therefore the lower the mass of the isobar, [sup.A.sub.Z]M, the higher is [Q.sub.b], i.e., the isobar with minimum nuclear mass is the most stable. For example, the mass of a set of isobars with odd mass number (A = 135) can be represented by a parabolic function of Z (Figure I-6 left). The stable nucleus [sup.135]Ba lies at the bottom of the parabola. The isobars on the neutron-rich side of the parabola (left arm) are unstable and will be transformed into [sup.135]Ba by successive negative s decays ([sup.A.sub.Z]M [right arrow] [sup.A.sub.Z+1][M.sub.+] + [e.sup.-] + [??], where [bar.v] is the antineutrino and [e.sup.-] is the electron). The isobars on the proton-rich side of the parabola (right arm) will again be transformed into [sup.135]Ba by successive positive s decays ([sup.A.sub.Z]M [right arrow] [sup.A.sub.Z-1][M.sup.-]+ [e.sup.+] + v, where v is the neutrino and [e.sup.+] is the positron) or by electron capture ([sup.A.sub.Z]M + [e.sup.-] [right arrow] [sup.A.sub.Z-1]M + v), i.e., anucleus captures an electron from the inner electron orbitals of the atom. Therefore this kind of parabola is often called the s-stability valley.
(Continues...)
Excerpted from A Compendium of Geochemistry by Yuan-Hui Li Copyright © 2004 by Princeton University Press. Excerpted by permission.
All rights reserved. No part of this excerpt may be reproduced or reprinted without permission in writing from the publisher.
Excerpts are provided by Dial-A-Book Inc. solely for the personal use of visitors to this web site.
Table of Contents
Preface xi
Chapter 1 Atoms, Nuclei, and Energy 3
Introduction 3
I-1. Periodic Table of the Elements and Electron Configurations of Atoms 3
I-2. Atomic Nuclei and Nuclear Binding Energies 13
I-3. Cohesive Energies among the Atoms of Pure Metals and Nonmetals 19
I-4. Ionization Energies and Electron Affinities of Gaseous Atoms and Ions 23
I-5. Ionic Radii and Ionic Potentials 28
I-6. Electric Polarizability 35
I-7. Electronegativity 39
I-8. Crystal Lattice Energies 44
I-9. Hydrolysis of Canons and Dissociation of Oxyacids 48
I-10. Solubility Products and Affinity of Aqueous Cations to Oxides 52
I-11. Concluding Remarks 53
Chapter 2: The Solar Nebula and Nucleosynthesis 55
Introduction 55
II-1. Elemental and Isotopic Compositions of the Solar Nebula 55
II-2. Cosmological Nucleosynthesis 63
II-3. Stellar Nucleosynthesis 68
II-4. Concluding Remarks 82
Chapter 3: Structure and Chemistry of the Solar System 83
Introduction 83
III-1. Motion of Interplanetary Objects 83
III-2. Asteroids and Comets 86
III-3. Planets, Their Satellites, and Their Rings 99
III-4. Condensation of Solid Dusts from the Solar Nebular Gas 107
Chapter 4: Distribution of Elements in Meteorites 119
Introduction 119
IV-1. Classification of Meteorites 119
IV-2. Oxygen Isotopes and Possible Genetic Relationships among Subclasses of Meteorites 129
IV-3. Bulk Compositions of Chondrites and Factor Analysis 133
IV-4. Cosmochemical Classification of Elements 144
IV-5. Matrices and Chondrules of Chondrites 154
IV-6. Ca-Al-Rich Inclusions in Chondrites 167
IV-7. Igneous Differentiation in Achondrites and Iron Meteorites 179
IV-8. Concluding Remarks 188
Chapter 5: Igneous Rocks and the Composition of the Earth 189
Introduction 189
V-1. Classification Scheme for Igneous Rocks 189
V-2. Earth's Structure and Mineral Composition 193
V-3. Partial Melting and Fractional Crystallization Models of Igneous Rocks 197
V-4. Deduction of the Primitive Upper Mantle Composition 209
V-5. Partition of the Elements between Mantle and Core 215
V-6. Continental and Oceanic Crusts 221
V-7. Relationship between the Compositions of Mantle and Crust 231
V-8. Isotopic Heterogeneity of the Mantle 234
V-9. Case Studies of Elemental Association in Igneous Rocks 242
V-10. Concluding Remarks 249
Chapter 6: Weathering and Sedimentary Rocks 253
Introduction 253
VI-1. Weathering of Igneous Rocks 254
VI-2. Dissolved Products of Chemical Weathering 260
VI-3. Major Classes of Sedimentary Rocks 264
VI-4. Relative Abundances of Major Sedimentary Rock Types and Mass Balance 268
VI-5. Shales and Related Materials 273
VI-6. Trace Elements in Sandstone and Limestone 283
VI-7. Iron Formations 288
VI-8. Partition of Elements between River-Suspended Particles and River Water, and the Adsorption Model 296
VI-9. Concluding Remarks 302
Chapter 7: Distribution of Elements in the Ocean 303
Introduction 303
VII-I. Concentrations of Elements in the Oceans 3(13
VII-2. Chemical Speciation of Elements in the Ocean 312
VII-3. Marine Algae and Plankton 317
VII-4. Zooplankton Fecal Pellets and Sediment Trap Material 325
VII-5. Marine Sediments 329
VII-6. Marine Manganese Nodules and Seamount Manganese Crusts 341
VII-7. Marine Phosphorite 347
VII-8. Hydrothermal Vents of the Mid-Ocean Ridges 348
VII-9. Concluding Remarks 352
Chapter 8: Biosphere and Homo Sapiens 355
Introduction 355
VIII-1. Are all Creatures Created Equal? 355
VIII-2. Human Body 366
VIII-3. Coals, Crude Oils, and Organic-Rich Shales 376
VIII-4. Relative Volatility of Elements and Compositions of Aerosol Particles 387
VIII-5. Effects of Fossil Fuel Burning on the Chemistry of Rain and River Waters: A Case Study 405
VIII-6. Concluding Remarks 411
Appendix: Rayleigh Condensation and Evaporation Models 413
Appendix Table A-1: Ionization Energies 415
Appendix Table A-2: Abundance of the Nuclides 418
Appendix Table A-3: Minerals in Meteorites 424
Appendix Table A-4: Minerals in Igneous Rocks 426
References 429
Index 465
What People are Saying About This
This book emerges at a time when the scientific community recognizes the need for geochemical reference data sets. Yuan-Hui Li couples an extremely wide range of topics with an in-depth treatment that is very close to state-of-the art. A Compendium of Geochemistry may be seen as a resource to researchers who wish to expand into other fields and as a complement to traditional geochemistry textbooks,one that is useful to students as well as teachers.
This book will fill an important niche in the field of geochemistry. The compilation covers an enormous amount of data and is a most valuable addition to the literature.
"This book will fill an important niche in the field of geochemistry. The compilation covers an enormous amount of data and is a most valuable addition to the literature."—Heinrich D. Holland, Harvard University"This book emerges at a time when the scientific community recognizes the need for geochemical reference data sets. Yuan-Hui Li couples an extremely wide range of topics with an in-depth treatment that is very close to state-of-the art. A Compendium of Geochemistry may be seen as a resource to researchers who wish to expand into other fields and as a complement to traditional geochemistry textbooks, one that is useful to students as well as teachers."—Hubert Staudigel, Scripps Institute of Oceanography, University of California, San Diego
This book will fill an important niche in the field of geochemistry. The compilation covers an enormous amount of data and is a most valuable addition to the literature.
Heinrich D. Holland, Harvard University
Recipe
"This book will fill an important niche in the field of geochemistry. The compilation covers an enormous amount of data and is a most valuable addition to the literature."Heinrich D. Holland, Harvard University
"This book emerges at a time when the scientific community recognizes the need for geochemical reference data sets. Yuan-Hui Li couples an extremely wide range of topics with an in-depth treatment that is very close to state-of-the art. A Compendium of Geochemistry may be seen as a resource to researchers who wish to expand into other fields and as a complement to traditional geochemistry textbooks, one that is useful to students as well as teachers."Hubert Staudigel, Scripps Institute of Oceanography, University of California, San Diego