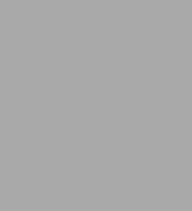
A Complete Manual of Amateur Astronomy: Tools and Techniques for Astronomical Observations
335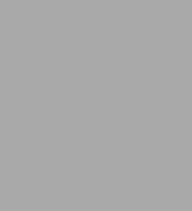
A Complete Manual of Amateur Astronomy: Tools and Techniques for Astronomical Observations
335eBook
Available on Compatible NOOK devices, the free NOOK App and in My Digital Library.
Related collections and offers
Overview
Product Details
ISBN-13: | 9780486152165 |
---|---|
Publisher: | Dover Publications |
Publication date: | 11/13/2012 |
Series: | Dover Books on Astronomy |
Sold by: | Barnes & Noble |
Format: | eBook |
Pages: | 335 |
File size: | 24 MB |
Note: | This product may take a few minutes to download. |
Read an Excerpt
A Complete Manual of AMATEUR ASTRONOMY
By P. CLAY SHERROD, THOMAS L. KOED
Dover Publications, Inc.
Copyright © 1981 Prentice-Hall, Inc.All rights reserved.
ISBN: 978-0-486-15216-5
CHAPTER 1
THE SELECTION OF A TELESCOPE
Getting that first telescope or the instrument you have been planning as your final investment is an exciting adventure. A new telescope can bring hours—even years—of enjoyment to the whole family. Moving up to a larger, more efficient instrument allows the serious amateur astronomer to engage in research, astrophotography, and make a contribution to the scientific field of astronomy.
However, in either case—for the beginner or the semiprofessional astronomer—the choice of a telescope is a serious matter and one that should be thoroughly planned prior to the final investment. As in many other endeavors, there is no one perfect telescope for general all-purpose, all-interest viewing. Many telescopes are well suited for studies of the planets, exhibiting very fine detail of Mars, Jupiter, and Saturn's magnificent ring system. Others show these objects too glaringly without enough built-in magnification to do much good, yet these instruments excel in astrophotography or in views of the star clouds stretched through the summer Milky Way. Picking up some popular astronomy magazine with its dazzling array of advertisements from commercial sources merely compounds the difficult question: "Which telescope is best suited for my interests?"
THE MANY FUNCTIONS OF AN ASTRONOMICAL TELESCOPE
The beginning astronomer is usually baffled by claims for "power," that is, magnification that will seemingly bring anything one wishes into view. Small department-store types of telescopes are often advertised as having such excessive magnifications as 454, 600, or even 1000. Such magnifications are not practical, even in the largest amateur telescopes. What, then, is the purpose of the telescope? It would seem at first that magnification is the primary purpose of the instrument, but there are more important functions to look for in choosing an instrument.
Light Grasp
Opinion varies, and much is already in print about the quality and performance of astronomical telescopes, but there is no substitution for size, that is, the diameter of the aperture of the primary optics. A larger telescope will exhibit more subtle detail in nebulous objects and show fainter stars because it simply has more surface area on which light is collected. Basically, before all other considerations, a telescope must be a light collector. This does not mean that instruments of smaller aperture are not suitable for astronomical studies, but only that in most cases added light grasp is an asset. The brightness of all celestial objects is designated on a somewhat arbitrary scale, known as the apparent magnitude scale, in which we assume that a star near the naked-eye limit—approximately magnitude 6—is is about 100 times fainter than a star of the magnitude 1. The basis for the assumption arose early in the second century BC when the astronomer Hipparchus compiled a star catalog containing about 1000 stars; each of the brightest stars of the major constellations were assigned first magnitude, whereas those just at the limit of naked-eye vision were said to be sixth magnitude. Consequently, a difference of five magnitudes corresponds to a ratio of 100:1, or–as suggested by astronomer Norman R. Pogson in 1856–each magnitude corresponds to the fifth root of 100, or 2.512. Put another way, a star of magnitude 1 is simply 2.512 times brighter than one of magnitude 2. It is somewhat confusing to those new to astronomy that the brigher stars have lower numbers than do the fainter ones. Exceptionally bright objects even have negative numbers to allow for the continuation of the Hipparchus/ Pogson concept.
Such magnitudes are known as apparent, or relative, because they serve only to intercompare stars and celestial objects as we perceive their brightnesses from earth. Obviously, a star appears quite bright to us if it is very close, and it appears fainter as it moves farther away. It is important to astronomers to understand the "real" or absolute magnitude of objects. How much light does a star actually emit into space? All stars emit light in relation to their size and temperature. By knowing the distance of the celestial object, we can compute the real brightness because the light we receive from the object varies inversely to the square of the distance that the object is from us.
Comparing the absolute magnitudes of stars allows astronomers to evaluate in more depth the physical nature of the processes of those stars. If we were able to establish just how bright each celestial object would appear if all were positioned at exactly the same distance from earth, the evaluations are simplified. Thus, the absolute magnitude of any celestial object is defined as the magnitude that that object would have if it were positioned at a distance of 10 parsecs in space, or about 32.6 light years. Our own star, the sun, shines with an apparent magnitude of -26.5, yet at that distance its absolute magnitude is a mere +4.85, near the limit of the naked eye, which is magnitude +6.
In most studies by amateur astronomers, references made to magnitude concern apparent magnitude—the brightness of the object as we see it in our instruments or with our naked eyes. The professional astronomer and theorist are more concerned with the absolute magnitude. Yet the apparent magnitude and the absolute magnitude can be derived each from the other. The first advantage of light grasp is that the limitingmagnitude of a telescope increases with increased aperture. We think of limiting magnitude in terms of the faintest star that is visible under the best conditions in any given aperture. Most tables that compare aperture to limiting magnitude seem somewhat conservative in my estimation. There is no doubt that the most acute eye is capable of perceiving a faint star just beyond another's view, but the values should be near constant for the majority of observers (See Table 1-1).
In very exacting situations, with trained observers, the magnitude limit of any telescope can be increased by adjusting the magnification upward slightly, thus increasing the threshold contrast of the object and the background sky.
The figures given in Table 1-1 can be misleading for persons not prepared to interpret them first. For example, even though a 25 cm (10-inch) reflector might be capable of showing stars to magnitude 14.2, there is no way the same telescope could be used visually to survey for galaxies of that same magnitude. When magnitudes of celestial objects are expressed, it is in terms of integrated light, that is, light compacted to a point source, like a star, and then measured. Thus, for example, the bright galaxy Messier 33 in Triangulum is a quite difficult object to view in a 25-cm telescope, even though its total brightness is greater than magnitude 6. Such extended objects will appear considerably brighter if their sizes are relatively small by comparison to Messier 33, whose light is spread out over an area greater than 1° of sky.
Increased aperture will likewise increase the resolution of an instrument, but atmospheric turbulence and differential cooling and heating of optics of larger telescopes will often cancel the resolution gained by an increase of objective diameter.
Resolution
Another important function of a telescope, resolution, is related not only to the size of the objective lens or mirror, but to the quality of the optics and the conditions under which they are used. According to Dawes' criteria of telescope resolution, the larger the diameter of a telescope's optics, the finer the detail that can be seen, as expressed in the formula:
sep" = 4.56/d
where seep" represents the minimum separation in seconds of arc of two stars of equal magnitude, at which each star is clearly seen to be distinct from the other in a given aperture. Dawes' criterion is 4.56, and d is the diameter of the telescope lens or mirror, in inches. Table 1-2 lists telescopes of various apertures and their resolving power, according to Dawes' limit.
The limits set forth by the foregoing equation seem to hold fairly well but only in instances of excellent visibility and steady air. If the air is quite turbulent, due to the rapid mixing of warm and cool air, no telescope can expect to achieve the performances given in Table 1-2. Likewise, only the finest optical surfaces can attain resolution close to the figures in Table 1-2. In addition, instruments of short focal length (f/6 or faster) generally cannot resolve nearly as well as telescopes of longer focal length. Consequently, we would expect the long-focus, clear-aperture refractors to excel in resolution—and they do, in cases reaching or exceeding Dawes' limit.
Resolution of an optical system is also affected by the bending and scattering of light that must happen through most reflector telescopes. In classical Newtonian and Cassegrainian telescopes the light as it enters the tube must pass across not only the secondary mirror but also the supporting mechanism (the "spider") for that mirror. In the catadioptic, or compound, telescopes, in which a front correcting lens supports the secondary mirror, the scattering of light is much less severe.
Magnification
If a telescope has great light-gathering power (large aperture) and good resolution (aperture + quality optics + air steadiness), it also should be well suited for magnification. As a matter of fact, in very steady conditions a magnification of 50 power per inch of aperture must be used to achieve optimum resolution. Anything more, and the image degrades rapidly. Even under the steadiest conditions, the eye is a limiting factor in maximum, and even minimum, magnification.
For example, under dark sky conditions, with the human eye totally "dark-adapted," the pupil should measure about 8 mm. A beam of parallel light wider than the eye's ability to receive it emerging from an eyepiece of very low power results in vignetting (i.e., loss of perception of the entire field of view). Thus, every telescope is ruled by the low-power law for minimum magnification. This law is expressed by the formula:
m[??] = aperture of telescope in mm/8 mm
where m[??] represents the lowest usable magnification for a particular instrument, and the aperture of the telescope is expressed in millimeters divided by 8 mm (the diameter of the pupil of the human eye when fully dark-adapted).
It has probably occurred to you at this point that there is most likely some criterion for determining the maximum power that can be utilized. The human eye achieves greatest resolution when the pupil is constricted to only 3 mm. This is because constriction causes the focal ratio of the eye to change from about f/2.7 to about f/7. Yet, when substituting 3 mm for the 8 mm of the fully dilated eye, the resulting values are still less than the highest useful magnifications. I have found, through practice, that the most practical upper limit of magnification is about five times the lower limit. Table 1-3 gives recommended lowest, optimum, and highest magnifications of telescopes of various aperture.
The upper limits of magnification on any given night, of course, will depend on the state of the atmosphere for that night. On the crisp cool nights when the sky is amazingly transparent, the steadiness of the air is usually quite poor. Conversely, when there is a heat inversion in the summer months, with stagnant air overhead, the air is usually quite steady, allowing for excellent views of the planets and double stars. Like resolution, the magnification potential of a telescope increases on such nights because the boiling of the earth's air is at minimum and definition is steady and uninterrupted.
Telescopes that have a minimal number of light obstructions in the optical path are potentially higher magnifiers than other types. Refractors, with their clear unobstructed aperture, usually can hold more magnification while maintaining steady images than can a reflector of equivalent size.
TELESCOPE DESIGN AND RECOMMENDED USE
Three basic types of telescopes are of interest to beginning and advanced amateur astronomers. All three types are capable—at least to some degree—of performing the functions of light grasp, resolution, and magnification. Any type of telescope can be used for almost every project described in the following chapters, but some are better suited for particular uses than others. The refractor telescope is that which is commonly brought to mind when someone mentions "astronomy." The refractor uses a lens as its light collector at one end, and an observer at the other. Its counterpart is the reflector, which uses a mirror, as its name implies. The third common telescope in amateur hands is the compound, or cotadioptic design, which uses both mirrors and lenses to provide long focal lengths and quality image formation. Each of the three types has certain advantages and disadvantages, but when properly used each gives endless enjoyment and is a tool whereby the projects described in the following chapters can be begun with scientific vigor.
The Refractor
The refractor telescope is what the layman envisions as a telescope: a long tube with a lens at one end and a human eyeball at the other. The front element, or objective, is a convex lens of long focal length (see Figure 1-1 a), followed by a second lens of equal size. Together the pair of lenses form an achromatic lens, one that should provide images in their true colors.
However, any piece of glass has prismatic capability, refracting various wavelengths of light in varying degrees, and even achromatic lenses are not free from some spurious violet coloration. The longer the focal ratio of the system (f/12 and longer), the less detrimental the color becomes. If it were not for the second, or flint lens, behind the convex glass (the crown lens), the spurious blue introduced through the aberration of light would render the instrument almost useless.
To produce an achromatic objective for a refractor requires an optician to grind, polish, and figure four surfaces, not just one, as in the case of a reflector telescope. Such work is time consuming and expensive; likewise, the pure glass, free of defects and bubbles, must be carefully and individually selected from expensive stock. The heart of the refractor—its objective lens—is therefore a very costly item.
Refractors become quite massive in the larger sizes. Even a 4-inch refractor has a tube 60 inches long and must be mounted high enough to allow the observer easy access beneath the eyepiece when the instrument is aimed overhead. Because of this requirement for rigidity to accommodate the long tube, the height of the instrument, and the size of the optical path, refractors must be mounted on much more massive mountings than a reflector of normal (f/8) focal ratio with an equivalent aperture. Figure 1-2 shows a 4-inch refractor in use. Transportation of refractors with apertures of 4 inches and greater also becomes quite a problem. If one does not have some type of observatory shelter in which to house the instrument, it must be disassembled each night following observing and taken back indoors. If one wishes to take the telescope to the mountains where the skies are darkest, the refractor is usually of prohibitive size. Nonetheless, the refracting telescope is still the favorite among many critical observers because it provides very dark background sky, large image scale, and superb resolution. I have even heard the comment, "I would surely rather look at the sky than to have to watch a reflection of the same thing." Perhaps there are still some aesthetics involved in amateur astronomy.
The first refractor used for astronomical observations to any degree was made by the famous Galileo Galilei in 1609. Through it he discovered the four major satellites of Jupiter, craters on the moon, sunspots, and the phases of Venus.
The refractor is an excellent choice for the discriminating observer of the moon, planets, and sun (instrumental requirements for those projects are discussed in Chapters 6, 8-10, and 5, respectively). The instrument in sizes 4 inches and larger provides crisp, sharp images of such objects, and is less subject to internal heating currents than open-tube instruments. The long focal length of the refractor is advantageous for planetary study, resulting in a large image scale with minimal eyepiece magnification.
(Continues...)
Excerpted from A Complete Manual of AMATEUR ASTRONOMY by P. CLAY SHERROD, THOMAS L. KOED. Copyright © 1981 Prentice-Hall, Inc.. Excerpted by permission of Dover Publications, Inc..
All rights reserved. No part of this excerpt may be reproduced or reprinted without permission in writing from the publisher.
Excerpts are provided by Dial-A-Book Inc. solely for the personal use of visitors to this web site.
Table of Contents
1. The Selection of a Telescope.2. Instrument Setup and Maintenance.
3. Observations of Meteors.
4. Comets: A Guide to Observation, Photography, and Discovery.
5. Amateur Studies of the Sun.
6. Lunar Topography.
7. Lunar Occultations.
8. Mars: The Red Planet.
9. Jupiter: An Amateur's Guide to Research Projects.
10. Observations of Saturn.
11. Visual Photometry of the Minor Planets.
12. Studies of Variable Stars.
13. An Introduction to Photoelectric Photometry.
14. Astrophotography for the Amateur Astronomer.
Glossary. Appendices. Index.