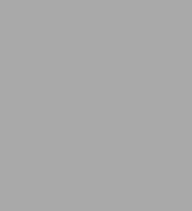
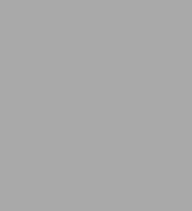
Hardcover
-
PICK UP IN STORECheck Availability at Nearby Stores
Available within 2 business hours
Related collections and offers
Overview
Thomas Kiørboe examines the life and interactions of plankton organisms with the larger aim of understanding marine pelagic food webs. He looks at plankton ecology and behavior in the context of the organisms' immediate physical and chemical habitats. He shows that the nutrient uptake, feeding rates, motility patterns, signal transmissions, and perception of plankton are all constrained by nonintuitive interactions between organism biology and small-scale physical and chemical characteristics of the three-dimensional fluid environment.
Most of the book's chapters consist of a theoretical introduction followed by examples of how the theory might be applied to real-world problems. In the final chapters, mechanistic insights of individual-level processes help to describe broader population dynamics and pelagic food web structure and function.
Product Details
ISBN-13: | 9780691134222 |
---|---|
Publisher: | Princeton University Press |
Publication date: | 09/22/2008 |
Pages: | 224 |
Product dimensions: | 6.10(w) x 9.30(h) x 0.80(d) |
Age Range: | 3 Months |
About the Author
Read an Excerpt
A Mechanistic Approach to Plankton Ecology
By Thomas Kiørboe Princeton University Press
Copyright © 2008 Princeton University Press
All right reserved.
ISBN: 978-0-691-13422-2
Chapter One INTRODUCTION
1.1 Biological Oceanography-Marine Biology-Ocean Ecology
LIKE SPORTS FISHING. I used to have a small boat from which I fished for herring, cod, and trout in the Øresund. Angling there can be quite productive, in particular if you learn where to go. At certain spots there are more cod than at others, for whatever reason. Once such spots were found, we used to find them again from land or sea marks. For example, 200 m north of a particular green buoy very often there would be cod. Eventually I knew so many "hot spots" that, whenever I went fishing, I would catch some cod. And eventually this lost its excitement. I sold the boat and started fishing for trout from the coast. Here the approach is not blind. You can see both the fish and its environment. You learn to know where to find the fish using relevant cues-that is, not a green buoy but a certain type of vegetation or structure of the seafloor. You learn to think as a trout; you develop intuition. When you visit new beaches, and if you are good enough, you can "read" the coast, and you can find fish. You can extrapolate the insights gained at one site to new, unknown locations.
Much of our knowledge of the biology of the oceans is derived from "blind" sampling. We use instruments to measure bulk properties of theenvironment, such as salinity and temperature, and we use bottle or net samples to extract knowledge about the organisms living in the ocean. This kind of approach has contributed important knowledge but has also influenced the way we view marine life. It leads us to focus on abundances, production rates, and distribution patterns. Such a perspective is very relevant in the context of the ocean as a resource for fisheries. It is also helpful in developing an understanding of biogeochemical issues such as ocean carbon fluxes. But on its own, this approach is insufficient, even for those purposes. The kind of intuition that we develop about marine life is, of course, influenced by the way we observe it, and because the ocean is inaccessible to us, and most planktonic organisms are microscopic, our intuition is rudimentary compared, for example, to the intuitive understanding we have about (macroscopic) terrestrial life. Our understanding of the biology of planktonic organisms is still based mainly on examinations of (dead) individuals, field samples, and incubation experiments, and even our sampling may be severely biased toward those organisms that are not destroyed by our harsh sampling methods. Similarly, experimental observations are limited to those organisms that we can collect live and keep and cultivate in the laboratory. One may argue that these limitations have biased our understanding of the ecology of the plankton and thus constrained our comprehension of the function of pelagic food webs (Smetacek and Pollehne 1986).
The ocean is structured on all spatial scales, but sampling averages over volumes, and this has led us to focus our attention on the potential importance of certain scales over others. In particular, sampling averages over volumes that exceed the ambit of individual plankters by orders of magnitude. Thus, we may relate organism distributions to distributions of salinity and temperature, for example, without knowing whether these are the cues to which the organisms respond. From sampling we can enumerate phytoplankton, zooplankters, and other particles, but we do not know how they are distributed relative to one another at a scale that is relevant to the organisms. We do not know whether the particles in a sample were originally aggregated as marine snow because such aggregates disintegrate to component particles when sampled by traditional means. In a water sample, we can also measure the concentrations of various solutes (dissolved organics, oxygen, nutrient salts), which may lead us to think that the solutes were homogeneously distributed in the sample before it was collected; they rarely are. And we may incubate water samples with radiotracers to get estimates of production rates of bacteria and phytoplankton. The implicit assumption is that such rates are representative of the corresponding rates in situ. But are they? These numbers, concentrations, and rates that are measured at scales that exceed the daily ambit of the individual organisms by orders of magnitude are insufficient to provide an understanding of how the organisms function in their environment. Visualization and observations as well as considerations at the level of the individual plankter are keys to establishing a mechanistic understanding of how the organisms function and interact and, hence, how the system of which they are part works (e.g., Azam and Long 2001).
Ecosystems consist of populations, which in turn consist of individuals that interact with one another and with the environment. Biological interactions in the ocean are not between populations or between trophic levels, as many box-model representations of pelagic food webs might lead us to think. Trophic levels and populations are abstractions, and interactions occur at the level of the individual. "Blind" sampling of bulk properties may result in observed distributional patterns, for example, that cannot be understood and explained from such an approach on its own. The picture must be complemented by approaches that consider the individual in its immediate environment and that provide a mechanistic understanding of the functioning of individuals and of components of the larger systems. This allows us to build models and to extrapolate observations beyond the system in which the observations were made.
Traditionally, scientists who go on cruises and examine distribution patterns of both biota and environmental properties using sampling are considered biological oceanographers, and those who explore the functioning of individuals, for example by conducting laboratory experiments with organisms, are considered marine biologists. We need to combine the two approaches to understand the ecology of the oceans. This book considers the functional ecology of planktonic organisms but with a view on how organism biology shapes the ecology of the oceans. In fact, as we proceed, and particularly by the end, we shall try to integrate our understanding of individual-level processes to predict properties of planktonic populations and of pelagic food webs.
The motivation to try to understand the ecology of planktonic organisms is twofold. The first driving force has to do with a simple interest in natural history. It is fascinating to watch the behavior of live plankters under the microscope or-better-free- swimming plankters by video; they have different but often beautiful forms and colors, and even closely related species may behave very differently, which makes identifying live plankton much easier than identifying dead ones. My own love for the plankton was elicited by one photograph in particular, of a very beautiful echinoderm larva, published in a popular book by Gunnar Thorson, and by the beauty of plankton in general (fig. 1.1). This fascination led me to a desire to understand why and how a plankter does what it does and how it solves various problems, among them, finding food in a dilute environment. The second reason for examining the adaptations and behavior of plankters is our interest in understanding overall properties of pelagic systems and how the pelagic system relates to the larger- scale issues of fisheries' yield, C[O.sub.2] balance, global climate, and others. Understanding the mechanistics of individual behaviors and interactions may allow us to predict rates and to scale rates to sizes, which, in turn, may help us understand the (size) structure and function of pelagic systems and to predict effects of environmental changes and human impacts.
1.2 The Encounter Problem
Life is all about encounters. In the ocean, for example, phytoplankton cells need to encounter molecules of nutrient salts and inorganic carbon; bacteria need to encounter organic molecules; viruses need to encounter their hosts; predators need to encounter their prey; and males need to encounter females (or vice versa). There is no life without encounters, and the pace of life is intimately related to the rate at which encounters happen. Other important processes in the ocean, such as the formation of marine snow aggregates, likewise depend on encounters, here encounters between the component particles. We are therefore interested in encounter rates. And we are interested in understanding the mechanisms that govern encounters. What are the constraints, and what are the implications of these constraints?
All organisms, including plankters, have three main tasks in life, namely to eat, to reproduce, and to avoid being eaten, all related to encounters or avoiding encounters. The behavior, morphology, and ecology of planktonic organisms must to a large extent represent adaptations to undertake these missions, and the diversity of form, function, and behavior that we can observe among plankters must be the result of different ways of solving the problems in the environment in which they live. The pelagic environment seen from the point of view of a small plankter is very different from the environment experienced by humans, and our intuition is often insufficient to allow us to understand the behavioral adaptations of planktonic organisms. Thus, although ornithologists to a large extent may be able to understand the behavior of their study organisms by using common sense, copepodologists rarely can, to rephrase the title of a classical ecology paper (Hutchinson 1951). For example, at the scale of planktonic organisms, the medium is viscous, and inertial forces therefore are insignificant, which makes moving an entirely different undertaking than what we as humans are used to or have seen other terrestrial animals do; the density of water is orders of magnitude higher than the density of air, which makes floatation easier and currents more important; for the smallest pelagic organisms (bacteria), thermally driven Brownian motion makes steering impossible; and most plankton use senses different from, and less far-reaching than, vision to perceive the environment. In addition, the pelagic environment is three-dimensional, whereas humans mainly move in only two dimensions. This implies, among other things, that average distances between a planktonic organism and its target may be very large, maybe thousands of body lengths. Because of the often nonintuitive nature of the immediate environment of small pelagic organisms, we need to appeal to fluid dynamic considerations in order to achieve a mechanistic understanding of the small-scale interactions between plankters and their environment.
In pursuing the encounter problem we can write a very general equation that describes encounter rates
E =[beta][ITLITL.sub.1][ITLITL.sub.2] (1.1a)
where E is the number of encounters happening per unit time and volume between particle types 1 and 2, [ITLITL.sub.1] and [ITLITL.sub.2] are the concentrations of these particles, and s is the encounter rate kernel ([L.sup.3][T.sup.-1]) (see table 1.1 for definition of symbols used). Often we are interested in looking at the per capita rate, that is, the rate at which one particle of type 1 encounters a particle of type 2:
e = E/ITL.sub.1] = s [ITLITL.sub.2] (1.1b)
For example, if particle 1 is a suspension-feeding ciliate and [ITLITL.sub.2] the concentration of its phytoplankton prey, then s is the ciliate's clearance rate, and e its ingestion rate (assuming that all encountered particles are ingested). The clearance rate is the equivalent volume of water from which the ciliate removes all prey particles per unit time. In many suspension-feeding ciliates, the clearance rate can be interpreted directly as a filtration rate; that is, the rate at which water is passed through a filtering structure that retains suspended particles. As a different but similar example: if particle 1 is a fish larva looking for food, and particle 2 its microzooplankton prey, then s is the volume of water that the larvae can search for prey items per unit time; if all encountered prey are consumed, then e is the ingestion rate of the fish larva. We may also see the process from the point of view of the prey, in which case s[ITLITL.sub.1] is the mortality rate of the phytoplankton or microzooplankton prey population through ciliate grazing or fish larval feeding. As a final example: if [ITLITL.sub.1] is the concentration of bacteria, and [ITLITL.sub.2] the concentration of organic molecules on which the bacteria feed, then e is the assimilation rate; it is more difficult to give a physical interpretation of s in this case. However, it is, like a clearance rate, the imaginary volume of water from which the bacterium removes all molecules per unit time. In fact, any encounter problem that I can think of can be cast in terms of the general equation (eq. 1), but obviously the interpretation or meaning of the terms may be very different.
The processes or mechanisms responsible for encounters are contained in the encounter-rate kernel. Obviously, from the examples above, these mechanisms are diverse. Intuitively, encounter rates depend on two factors: the motility of the encountering "particles" and the ambient fluid motion that may enhance encounter rates. Motility encompasses here the diffusivity of molecules, the swimming of organisms, and the sinking of particles. In regard to planktonic organisms, ambient fluid motion essentially means turbulence because planktonic organisms (contrary to benthic ones) are embedded in the general flow. From this consideration, one can see that there may be different components entering into the encounter-rate kernel depending on the specific problem under consideration.
The encounter problem-and equation 1-will be the primary issue throughout most of this book and of chapters 2-5 in particular. We shall examine the encounter problem for a number of fundamental processes in the plankton (e.g., feeding, mating, coagulation). And for each of the processes considered, we shall try to write simple models for s, as far as possible based on first principles. My presentation is case driven, but wherever possible or necessary, I will make excursions to more general explanations.
The purpose of this book is twofold. First and foremost, I want to explore the ecology of plankton organisms at the level of the individual by examining how they are adapted to the viscous, three-dimensional, and dilute (in terms of food) environment in which they live. My goal is to provide a mechanistic understanding of the functioning of individual plankters, both in terms of the interactions between the organisms and their immediate environment and in terms of the interactions between individuals. These are the topics of chapters 2-6. Second, I want to use the insights into individual-level processes to examine population- and ecosystem-level processes and patterns. Biological processes in the plankton occur at the level of the individual, and biological interactions in the ocean are between individuals rather than between populations or trophic levels; the latter are abstractions. Although the structure and function of pelagic food webs cannot be derived solely from a mechanistic understanding of the functioning of the individuals, important properties of distribution, population, and community patterns, and of the turnover of matter and energy in the plankton can be predicted from individual-level processes. This extension is the topic of chapters 7 (population processes) and 8 (pelagic food webs). The intention of these two final chapters is to illustrate the usefulness of combining oceanographic and biologic approaches to examine ocean ecology rather than to provide a complete description of pelagic ecosystems. Overall, throughout this book, emphasis will be on telling a coherent rather than a comprehensive story, and there are therefore many aspects of plankton ecology that are not covered here.
(Continues...)
Excerpted from A Mechanistic Approach to Plankton Ecology by Thomas Kiørboe
Copyright © 2008 by Princeton University Press . Excerpted by permission.
All rights reserved. No part of this excerpt may be reproduced or reprinted without permission in writing from the publisher.
Excerpts are provided by Dial-A-Book Inc. solely for the personal use of visitors to this web site.
Table of Contents
List of Illustrations ix
List of Tables xiii
Preface xv
CHAPTER ONE: Introduction 1
1.1 Biological Oceanography--Marine Biology--Ocean Ecology 1
1.2 The Encounter Problem 4
1.3 This Book 8
CHAPTER TWO: Random Walk and Diffusion 10
2.1 Random Walk and Diffusion 10
2.2 Example: Bacterial Motility 14
2.3 Fick's First Law 17
2.4 Diffusion to or from a Sphere 18
2.5 Feeding on Solutes 20
2.6 Maximum and Optimum Cell Size 22
2.7 Diatoms: Large yet Small 24
2.8 Diffusion Feeding 26
2.9 Non- Steady- State Diffusion: Feeding in Nauplii 28
2.10 Bacteria Colonizing a Sphere 30
2.11 Effect of Shape 31
2.12 Flux from a Sphere (or a Point Source): Chemical Signals 32
CHAPTER THREE: Diffusion and Advection 35
3.1 Moving Fluids 35
3.2 Viscosity, Diffusivity, Re, and Pe 35
3.3 Flow around a Sinking Sphere 37
3.4 Mass Transport to a Sinking Sphere 39
3.5 Example: Oxygen Distribution around a Sinking Sphere 40
3.6 Examples: Osmotrophs, Diffusion Feeders, and Bacterial Colonization of Sinking Particles 43
3.7 Eff ect of Turbulence on Mass Transport: Re, Pe, and Sh for Turbulence 45
3.8 Marine Snow Solute Plumes: Small- Scale Heterogeneity 49
3.9 The Chemical Trail: Mate Finding in Copepods 50
CHAPTER FOUR: Particle Encounter by Advection 57
4.1 Direct Interception versus Remote Detection 57
4.2 Particle Encounter by Direct Interception: Flagellate Feeding 58
4.3 Bacteria Colonizing Particles Revisited: Comparison of Encounter Mechanisms 60
4.4 Direct Interception: Coagulation and Marine Snow Formation 60
4.5 Remote Prey Detection: Encountering Prey in Calm Water 67
4.6 Turbulence and Predator- Prey Encounter Rates 69
4.7 Example: Feeding of the Copepod Acartia tonsa in Turbulence 72
4.8 When Is Turbulence Important for Enhancing Predator-Prey Contact Rates? 74
4.9 On the Downhill Side: Negative Eff ects of Turbulence on Predator-Prey Interactions 75
4.10 Encounter Rates and Motility Patterns: Ballistic versus Diffusive Motility 77
CHAPTER FIVE: Hydromechanical Signals in the Plankton 83
5.1 Copepod Sensory Biology 83
5.2 Decomposition of a Fluid Signal: Deformation and Vorticity 85
5.3 Signal Strength: Prey Perceiving Predator 87
5.4 Signal Strength: Predator Perceiving Prey 88
5.5 To What Flow Components Does a Copepod Respond? 89
5.6 Sensitivity to Hydrodynamic Signals 91
5.7 Predator and Prey Reaction Distances: Generation of a Hydrodynamic Signal 91
5.8 Attack or Flee--the Dilemma of a Parasitic Copepod 95
5.9 Maximal Signals, Optimal Sensitivity, and the Role of Turbulence 96
5.10 The Evolutionary Arms Race 98
CHAPTER SIX: Zooplankton Feeding Rates and Bioenergetics 101
6.1 Functional Response in Ingestion Rate to Prey Concentration 101
6.2 Example: The Functional Response in Oithona davisae 104
6.3 Other Functional Responses 105
6.4 The Components of Predation: Prey Selection 107
6.5 Prey Switching 113
6.6 Bioenergetics: Conversion of Food to Growth and Reproduction 113
6.7 Specific Dynamic Action: Egg Production Effi ciency in a Copepod 115
6.8 Scaling of Feeding and Growth Rates 117
6.9 Feast and Famine in the Plankton 118
CHAPTER SEVEN: Population Dynamics and Interactions 122
7.1 From Individual to Population 122
7.2 The Dynamics of a Single Population: Phytoplankton Blooms 123
7.3 Phytoplankton Population Dynamics and Aggregate Formation 125
7.4 Phytoplankton Growth and Light Limitation 127
7.5 Scaling of Growth and Mortality Rates 128
7.6 Populations with Age Structure: Life Tables 130
7.7 Behavior and Population Dynamics: Critical Population Size and Allee Eff ects 133
7.8 Life- History Strategies 135
7.9 Interacting Populations 140
7.10 From Individual to Population 149
CHAPTER EIGHT: Structure and Function of Pelagic Food Webs 151
8.1 Two Pathways in Pelagic Food Webs 152
8.2 Light and Vertical Mixing: Conditions for Phytoplankton Development 154
8.3 Bud getary Constraints: Nutrient Input and Sinking Flux 155
8.4 Cell Size, Water-Column Structure, and Nutrient Availability: Empirical Evidence 158
8.5 Cell Size and Nutrient Uptake 161
8.6 Cell Size, Turbulence, and Sinking 162
8.7 Cell Size, Turbulence, and Light 164
8.8 Why Are Not All Phytoplankters Small? The Signifi cance of Predation 165
8.9 Hydrodynamic Control of Pelagic Food- Web Structure: Examples 166
8.10 Species Diversity: The Paradox of the Plankton 170
8.11 Fisheries and Trophic Effi ciency 173
8.12 Fertilizing the Ocean--Increasing the Fishery and Preventing Global Warming? 177
References 183
Index 205
What People are Saying About This
This book allows scientists who work in any corner of the planktonic encounter arena to expand their views and teaching capabilities to cover the entire area. It will be the text of choice for graduate seminars in all the major and minor oceanographic and limnological institutions.
Peter A. Jumars, University of Maine
"A considerable achievement, this book is a vital addition to the field and an important tool in assisting interdisciplinary investigations in ocean biology."—Susanne Menden-Deuer, Princeton University"This book allows scientists who work in any corner of the planktonic encounter arena to expand their views and teaching capabilities to cover the entire area. It will be the text of choice for graduate seminars in all the major and minor oceanographic and limnological institutions."—Peter A. Jumars, University of Maine
A considerable achievement, this book is a vital addition to the field and an important tool in assisting interdisciplinary investigations in ocean biology.
Susanne Menden-Deuer, Princeton University