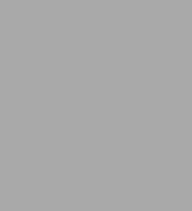
A Survey of Aircraft Structural-Life Management Programs in the U.S. Navy, the Canadian Forces, and the U.S. Air Force
112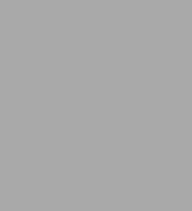
A Survey of Aircraft Structural-Life Management Programs in the U.S. Navy, the Canadian Forces, and the U.S. Air Force
112Paperback
-
SHIP THIS ITEMTemporarily Out of Stock Online
-
PICK UP IN STORE
Your local store may have stock of this item.
Available within 2 business hours
Related collections and offers
Overview
Product Details
ISBN-13: | 9780833038623 |
---|---|
Publisher: | RAND Corporation |
Publication date: | 05/15/2006 |
Pages: | 112 |
Product dimensions: | 6.06(w) x 8.67(h) x 0.30(d) |
Read an Excerpt
A Survey of Aircraft Structural-Life Management Programs in the U.S. Navy, the Canadian Forces, and the U.S. Air Force
By Yool Kim Stephen Sheehy Darryl Lenhardt
Rand Corporation
Copyright © 2006 RAND CorporationAll right reserved.
Chapter One
Introduction
The Air Force's Aging Fleets
The U.S. Air Force currently owns and operates approximately 6,000 aircraft to meet its force requirements. Because of budget pressures and high replacement costs, the Air Force is replacing its aircraft at a much slower rate than it did in the 1970s and the 1980s; thus, many old aircraft remain in the force (Pyles, 2003).
Table 1.1 lists some of the fleets that are the backbone of the force. Many aircraft currently in operation have been in service for more than 30 years with no retirement plans. Additionally, current plans indicate that many fleets will continue to be in service for many years. For instance, the B-52 fleet is expected to remain in operation for more than 80 years (Hebert, 2003). Although replacements have been identified for some of these fleets, a significant portion of them will not be replaced for many years to come because they are so large.
Fleet-Management Challenges with Aging Fleets
Sustaining the aircraft structure is one of the main challenges in extending use of old aircraft. As aircraft age and as their use increases, damage accumulates in their structures and various subsystems. Various damage mechanisms, such as fatigue cracking, corrosion, and stress-corrosion cracking,contribute to the deterioration of aircraft structure. Other nonstructural systems, such as the wiring system, also age as materials deteriorate from environmental exposure and mechanical stresses. Many older aircraft are facing these aging issues, and many more aircraft are expected to encounter them as the Air Force keeps them in service for many years.
Structural deterioration can lead to serious problems. Structural failure can have serious consequences, including loss of aircrews. There are growing concerns about a flight safety risk because of the increasing number and extent of structural deteriorations as aircraft age and with increasing use (Pyles, 2003; Hebert, 2003). There are further concerns about increasing demand for inspections, repair, and modifications. Such increases in maintenance workload may become extremely costly and may lead to poor aircraft availability, threatening mission capabilities (Hebert, 2003; Jumper, 2003; Secretary of the Air Force, 2002). In the past, the Air Force has imposed flight restrictions and grounded aircraft for the sake of aircraft safety after serious fatigue damage has been found (Pyles, 2003).
The Air Force thus faces the fleet-management challenge of converting these increasing structural needs to resource requirements, such as maintenance, modification, and replacement plans, to prevent structural failure, future economic burden, and loss of mission capability.
The U.S. Air Force Aircraft Structural Integrity Program
A series of catastrophic wing structural failures on B-47s (see the appendix) drove the Air Force to establish its ASIP in 1958. ASIP's overarching objective is to prevent loss of operational effectiveness and minimize costs while maintaining structural safety (i.e., preventing catastrophic structural failure) (AFPD 63-10).
ASIP provides a framework for ensuring the structural integrity of an aircraft throughout its life, from acquisition to retirement, and outlines the basic principles of structural-life management. During the acquisition phase, ASIP activities involve design, analysis, and tests to ensure that the aircraft structure is adequate to operate as intended. During the sustainment phase, ASIP activities involve the data collection, analysis, and tests needed to plan such continuous sustainment activities as maintenance and modifications to ensure that the structure remains safe until retirement. These activities provide information about structural conditions that can aid fleet-management decisions, such as creating inspection and maintenance plans, setting modification priorities, and enabling the decisionmakers to consider structural safety, cost, and operational effectiveness properly in managing the fleet.
The Air Force has been successful at ensuring structural safety for decades through ASIP. ASIP is a key contributor to the force-management processes, and the program's viability will be critical as the Air Force continues to operate an aging force to meet the nation's needs.
Current ASIP Challenges
In recent years, concern has arisen about ASIP's ability to continue meeting the Air Force's needs, given the aging force, budget pressures, and diminishing control of the servicewide ASIP (Tiffany et al., 1997). These factors are interdependent and together strongly influence the program's effectiveness.
Because the Air Force plans to fly many of its aircraft for many years, there is an increasing demand for more accurate knowledge about the current and future structural conditions of individual aircraft and the associated risks of structural failure. The need for engineering capabilities, both in terms of research and development and in terms of engineering analysis, is increasing as age-related problems grow. A 1997 National Research Council (NRC) study on the Air Force's aging force (Tiffany et al., 1997), as well as the engineering community at recent ASIP conferences, has voiced a concern that budget pressures, rather than structural needs, are driving the level of ASIP implementation because fleet managers must allocate available resources among multiple elements, such as sustainment of aging airframes and other aging aircraft subsystems (e.g., modernization of avionics).
Furthermore, control of ASIP has been diminishing over the years as a result of reforms in the 1990s to minimize government acquisition regulations. Prior to these acquisition reforms, Air Force Regulation (AFR) 80-13 and the ASIP standard, MIL-STD-1530, were used to enforce ASIP. The regulation ensured compliance with ASIP within the Air Force. The military standard required contractor compliance because it could be included in the contracts. However, with the acquisition reform, the AFR was converted to an Air Force Instruction (AFI) and the ASIP military standard (MIL-STD-1530) to a military handbook (MIL-HDBK-1530B). That change meant it could no longer be cited as a contractual requirement. As a result, the industry and the contractors, as well as the system program offices that carry out the ASIP, interpreted the former requirements as guidelines.
At the same time, other ASIP oversight activities have diminished. There have been concerns that the reduced authority and lack of oversight could lead to undesirable variability in ASIP implementation (omitted or incomplete ASIP tasks), especially in light of budget pressures.
These concerns about ASIP are compounded by the challenges the engineering community currently faces in communicating with decisionmakers. At recent ASIP conferences, the engineering community has expressed that one of the main challenges in structural-life management processes has been communicating structural integrity issues to decisionmakers. Community members cited several potential causes, including the following (ASIP Conference, 2003):
lack of technical understanding by the decisionmakers
insufficient data on structural conditions
lack of resources to gather sufficient information on structural conditions
lack of an outlet for communicating key structural integrity issues to the decisionmakers.
As a result, decisionmakers may not fully understand the fleets' structural conditions or the consequences of inadequate ASIP implementation.
The decisionmakers, on the other hand, may not be concerned about ASIP's effectiveness, since there have been no catastrophic structural failures in recent years. However, the concern is that the reduction in ASIP regulation, communication challenges, and budget pressures may lead to loss of control of the program as a whole, lack of awareness of structural conditions, and resource allocation challenges for those managing structural life. As a result, ASIP may not be implemented adequately (e.g., omission of or incomplete ASIP tasks), degrading its effectiveness.
These challenges, and the Air Force's need to continue to operate an aging force for many years to come, raise concerns about the future effectiveness of ASIP and the effects that may have on the force's operational effectiveness, aircraft safety, and sustainment costs.
The Air Force has begun to address some of these challenges. For example, in February 2004, the Aeronautical Systems Center's Engineering Directorate (ASC/EN) converted MIL-HDBK-1530B back to a military standard so that it could once again be used as a contractual requirement. This will reestablish some standardization of and control over ASIP. Further, the Air Force is in the process of institutionalizing a framework for structural risk assessment to enable communication of structural risks to decisionmakers, which will help them better understand structural integrity issues.
Study Purpose and Scope
The purpose of this research is to provide insights and guidance on how the Air Force can continue to strengthen and improve ASIP to meet the program's objectives in the presence of current challenges and needs.
Although some structural-life management issues that arise during the sustainment phase may be due to choices made during the acquisition phase (e.g., selection of material for performance rather than durability), this report focuses primarily on the sustainment phase to address current ASIP challenges for the aging force. The research scope is thus limited to aircraft that are no longer being procured.
The technical scope of ASIP is expanding to address other issues related to structural integrity, such as composite materials and corrosion. This report, however, focuses on ASIP processes that deal with metal fatigue.
Postulating that the regulations, communications between the structural-life management authorities, and the level of resources available influence the effectiveness of ASIP, we surveyed aircraft structural-life management programs in other military services to broadly understand their approaches to structural-life management, focusing on regulation, communication, and resource management.
The effects of these approaches on ASIP effectiveness are difficult and complex to quantify because of the large scope of the program and the effects of multiple variables, such as operational factors, technical scope, and the multiple elements involved in fleet management (e.g., depot organizations). This research is limited to a qualitative analysis of the implications of the different approaches to gain insights on how the Air Force can strengthen ASIP.
Approach
We believe that three interdependent factors promote adequate and effective ASIP implementation: (1) independent and balanced regulation, (2) clear and timely communications, and (3) adequate and stable resources (Figure 1.1). We therefore sought insights from other military aircraft fleets by identifying the mechanisms or approaches they use in these areas.
Selection Method and Data Collection
We selected the structural-life management programs in the U.S. Navy and the Canadian Forces for a comparison with that of the U.S. Air Force. The U.S. Navy was selected primarily because of its different technical approach to aircraft design and its sustainment concept. Additionally, the U.S. Navy is subject to U.S. Department of Defense (DoD) policies and budget pressures. We had learned that the Canadian Forces took a strikingly different approach (with a strong regulatory aspect) to structural-life management. Researching these services was convenient for data collection and provided a broad enough perspective on a variety of approaches for research purposes.
We collected information on the processes, organizational structure, policies, and technical methods of the three aircraft structural-life management programs. This information came from site visits, discussions at ASIP conferences, the literature, and service publications on policies and procedures. We did not collect any financial data and thus did not conduct cost analyses for comparison.
Potential Bias
The limited scope of the presented research focused our attention on factors that have been raised as key issues for the Air Force in recent years. We specifically sought ways to address those issues. As a result, there may appear to be a bias against the Air Force's approach. However, this research does not attempt to identify the relative effectiveness of the programs compared. We sought to minimize the potential for bias by identifying the potential positive and negative implications of all three approaches. Additionally, we tried to gain a full understanding of the potential effectiveness of each approach by assessing it within the contexts of the specific program and the service's operations.
Report Organization
Chapter Two begins by describing the technical basis for aircraft structural-life management programs, which affects various aspects of the program, then outlines the basic elements of such a program. The next three chapters describe each service's structural-life management program in turn: the U.S. Navy's program in Chapter Three, the Canadian Forces' program in Chapter Four, and the U.S. Air Force's program in Chapter Five. Each discussion includes operational factors; the program's structure and principal elements; and an explanation of how regulations, communications, and resource management come into play. Chapter Six makes some observations about the different approaches, summarizing each. Chapter Seven presents possible actions the U.S. Air Force could take to strengthen its ASIP.
Chapter Two
Background
This chapter begins with a background of aircraft structural design concepts, specifically those related to fatigue, and how they affect structural-life management. We then describe the basic elements of an aircraft structural-life management process. Different fatigue design concepts lead to different approaches to the elements of aircraft structural-life management, such as inspection and maintenance plans.
Fatigue as a Limiting Structural-Life Factor
Fatigue is one of the primary mechanisms causing deterioration of an aircraft's structure during its lifetime. Landings, takeoffs, and maneuvers subject the structure of an aircraft to repeated stress over its lifetime. This is referred to as cyclic or alternating loading (Figure 2.1). Cyclic loading causes fatigue cracks to form in the structure. These cracks grow longer with each stress cycle, degrading the aircraft's structural strength. A crack initially grows slowly, but the rate accelerates as these cycles accumulate, to the point at which rapid crack growth results in a fracture (i.e., the part breaks). Thus, one of the key design criteria for an aircraft is that it can endure accumulated fatigue damage over its service life to prevent structural failure.
Fatigue-Design Concepts: Damage Tolerance Versus Safe-Life
There are two distinct design approaches for protecting an aircraft's structure from failure due to fatigue damage: safe life and damage tolerance (Figure 2.2). These fatigue design approaches differ in how they model damage growth, what they assume about the initial conditions of materials, and the failure criteria they use to establish the aircraft's original design service life.
The fundamental difference between these is that the safe-life approach assumes that no fatigue cracks will exist in the structure during the specified lifetime for safe operation; the damage-tolerance approach assumes that potential fatigue cracks may exist in critical locations in fracture-critical parts. For practical purposes, the safe-life approach does assume that very small fatigue cracks may exist. The damage-tolerance approach requires that the structure be able to tolerate slowly developing cracks safely, until they can be detected and repaired.
(Continues...)
Excerpted from A Survey of Aircraft Structural-Life Management Programs in the U.S. Navy, the Canadian Forces, and the U.S. Air Force by Yool Kim Stephen Sheehy Darryl Lenhardt Copyright © 2006 by RAND Corporation. Excerpted by permission.
All rights reserved. No part of this excerpt may be reproduced or reprinted without permission in writing from the publisher.
Excerpts are provided by Dial-A-Book Inc. solely for the personal use of visitors to this web site.