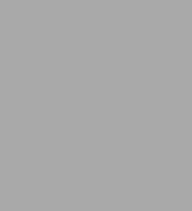
Aerobic Granular Sludge
181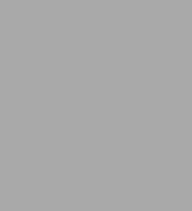
Aerobic Granular Sludge
181Paperback
-
SHIP THIS ITEMTemporarily Out of Stock Online
-
PICK UP IN STORE
Your local store may have stock of this item.
Available within 2 business hours
Related collections and offers
Overview
Product Details
ISBN-13: | 9781843395096 |
---|---|
Publisher: | IWA Publishing |
Publication date: | 03/31/2005 |
Series: | Water and Environmental Management Series (WEMS) |
Pages: | 181 |
Product dimensions: | 6.12(w) x 9.25(h) x 0.75(d) |
Read an Excerpt
CHAPTER 1
The Unity of Biofilm Structures
M.C.M. van Loosdrecht, M.K. de Kreuk and J.J. Heijnen
Department of Biotechnology, Delft University of Technology, Julianalaan 67, 2628BC, Delft, The Netherlands (E-mail: m.dekreuk@tnw.tudelft.nl; m.c.m.vanloosdrecht@tnw.tudelft.nl)
Abstract The morphology of biomass structures has been studied in many different ways for the last decades. However, the complexity of biofilm or granular structures makes it difficult to find cause and effect in questions concerning morphogenesis. With all stated research questions, one can ask if it is the microbiology or the process conditions that determines the structure of the aggregates. In this paper, biofilm, aerobic granular sludge, and bulking sludge experiments, as well as biofilm modeling studies, are presented to show that process conditions are of major importance to determine the final biofilm structure.
Keywords: Aerobic granular sludge, biofilm, growth rate, process parameters, shear
Introduction
Over the past ten years, much research has been performed in the field of aerobic granular sludge. This research has two reasons: first studies are performed because of a general interest in background and morphogenesis of bacterial structures. Secondly, granular sludge has the potential to form the base of a new compact wastewater treatment system.
Morphology of biomass structures is studied in many different ways: syntrophic relations of bacteria in anaerobic granular sludge studies, presence of specific organisms in bulking sludge, and the role of the microorganisms in building the porous structure of biofilms. Behind all these research questions, one can ask if it is the microbiology or the process conditions that are decisive for structuring the morphology.
In general biofilm structures are highly complex ecosystems, according to microbiology, morphology and flow. In these systems, the structure of the biofilm influences the activity (position of different organisms, porosity), and the activity of the organisms influences the structure of the biofilm. Due to this complexity of biofilm structures, it is very difficult to find cause and effect in questions concerning the morphogenesis. It is difficult to get representative samples from most biofilm processes. For example, how many granules should be analyzed by a microelectrode or sliced for FISH analysis before a statistically significant conclusion can be drawn about the average position of different groups of organisms in a structure? It is also very difficult to change only one experimental parameter in biofilm experiments. As an example, changing a concentration causes changed fluxes, penetration depth, biological activity and eventually granule structure. In this case, modeling can be used in order to evaluate efficiently the relative importance of parameters on the biofilm or granule system.
Biofilm Complexity
From experiments in existing biofilm systems as expended granular sludge bed (EGSB) reactors, Internal Circulation (IC) reactors and Biofilm airlift systems, it can be seen that three different factors lead to the same change. An increased surface loading rate, a decreased shear force and an increased potential growth rate of the microbial population all lead to thicker, less dense and more porous biofilms/granules. Additionally, it has been shown in steady-state systems that a large part of detaching biomass is growth related, not shear related as generally assumed (Van Loosdrecht et al., 1995). Experiments also showed that the redox gradient forms a more important parameter in granule structure than the growth rate gradient. In other words, biofilm structures are primarily affected by the concentration of the electron acceptors, and secondly by the maximum growth rate of the specific microbial populations. For example, anaerobic organisms can only grow in the anaerobic core of a granule (lowest redox), while their actual growth rate can be higher than the nitrifying organisms that are more on the outside in the granule at high redox potentials.
The general hypothesis of the formation of smooth and dense biofilms that was postulated is: the lower the actual growth rate and the higher the shear stress, the smoother the biofilms will become (Van Loosdrecht et al., 1995, 1997) (Figure 1).
Lessons from Modelling
As mentioned above, biofilm models can help to understand the importance of different parameters. In the 1970's, biofilm modeling started with a 1- dimensional model, in which a uniform biomass was defined with a substrate gradient in the biofilm. This model was extended to a multi-species and multi-substrate biofilm model, which was still 1D. In the late 1990's, 2-D and even 3-D multi-species and multi-substrate biofilm models were developed, in which the change of substrate concentration, growth rate and biomass density and behavior is determined for every biofilm domain in the modeled reactor. In this model, different relations for the main processes are solved:
1) Laminar flow equations according to Navier-Stokes relations;
2) Diffusion, convection and reaction in mass balances of all available substrates;
3) Mass balances of the biomass, growth;
4)Discrete or continuous biomass spreading, in which biomass shifts to a different position when the actual particle concentration exceeds the maximum particle concentration in a certain domain;
5) Mechanical equilibrium equations determining the stresses and strains on the biofilm;
6) Discrete and continuous biofilm detachment.
Different models based on different calculatory procedures (cellular automata, continuum, and individual particles) were developed, all leading to a good description of the experimental observations that a fingertype outgrowth of the biofilm forms at increased substrate gradients in the biofilm or increased substrate limitation (Figure 2). The main conclusion that can be drawn from biofilm modeling results, so far, is that the morphology of the biofilms are a result of interaction between growth velocity of the biofilm front, depending on growth rate and loading rate, and the shear rate. Furthermore, there is no need to assume specific microbiological processes influencing biofilm morphology in order to explain the present observations of biofilm systems.
Applications to Granular Sludge
Lessons from biofilm experiments and models show that there are different circumstances under which granule formation can be expected. First, sufficient shear is needed in relation to the maximal growth rate of the ty-pe of organisms (methanogens versus acidogens, nitrifiers versus heterotrophs; the latter groups will form less dense structures under the same circumstances as the first groups and would require higher shear rates). Second, high substrate concentrations are needed, in order to prevent strong gradients inside the granule. This can be obtained in a plug-flow or SBR process. Third, selection for slow growth is necessary (as obtained by applying a feast-famine regime if needed). For aerobic granule formation, this last condition seems to be crucial. This can be met by the conversion of readily degradable COD in slowly degradable substances, as internal storage polymers. To convert all available easy degradable COD, phosphate or glycogen accumulating organisms should be selected. Research showed that this leads so far to the most stable aerobic granules under unfavorable circumstances at low dissolved oxygen concentrations and low shear stress (De Kreuk and Van Loosdrecht, 2004).
One question that rises from the aerobic granular sludge research is if bulking sludge is the other side of the same medal. In other words, are the same mechanisms responsible for the existence of filamentous bulking sludge flocs as the mechanisms responsible for aerobic granule formation? From bulking sludge research it was found that the specific maximum substrate uptake rates in bulking sludge are more or less equal to the specific maximum substrate uptake rates of well settling sludge (-qsmaxbulking sludge ≈ -qsmaxwell settling sludge).
Furthermore, the specific PHB production rate of the two types of sludge is also more or less similar (qpmaxbulking sludge ≈ qpmaxwell settling sludge) (Martins et al., 2004). These two observations contradict the conventional kinetic selection theory and underlines that the substrate concentration in bulking sludge phenomena is important as well. Experiments show that with decreasing substrate concentration and therefore decreasing substrate uptake rates, activated sludge flocs become more and more irregular. When the substrate uptake rate is bigger than 0.8 times the maximum substrate uptake rate granules with a diameter larger than 0.5 mm will form and that only a few filaments exist. With substrate uptake rates between 0.6 and 0.8 times the maximum uptake rate, fingertype structures come into existence and flocs become more porous, while still few filaments are present. Substrate uptake rates below 0.6 times the maximum uptake rate lead to filamentous outgrowth and fingertype structures (Figure 3) (Martins et al., 2003).
Unity of Biofilm Structures
Experiments and modeling of bulking sludge, granule formation and biofilms led to the same conclusions towards structure and morphology. It can be said that these different morphologies of microbial structures are outings of the same phenomena and can be explained from the same basic principles. In conclusion, it can be stated that there is no need for theories based on specific microbial processes and that process conditions are of major importance for the final structure.
CHAPTER 2
Comparison of the granulation of activated sludge grown on carbohydrate- and protein-rich wastewaters
Han-Qing Yu, Yu-Ming Zheng, Kui-Zu Su
Abstract Granular activated sludge was cultivated in two sequencing batch reactors fed respectively with a synthetic sucrose-rich wastewater and a soybean-processing wastewater. The formation of granular activated sludge in the two reactors and their physicochemical characteristics were compared. With the granulation, the settling ability of the sludge continuously improved, as evidenced by a decreased sludge volume index and an increased settling velocity. The mature granular sludge was nearly spherical and had a strong structure. The granular activated sludge in the two reactors had similar physicochemical characteristics. This suggests that the substrate component was not a crucial factor for the granulation of activated sludge. The data reported in this study provided useful information about the development of aerobic-granule-based bioreactors for the treatment of carbohydrate- and protein-laden wastewaters.
Keywords Activated sludge; Carbohydrates; Granulation; Sequencing batch reactor (SBR); Soybean-processing wastewater; Sucrose
Introduction
After the original activated sludge process was invented, various modified processes have been developed and applied to treatment municipal and industrial wastewaters. Since the performance of the activated sludge processes is limited by the capacity of a clarifier for the separation of the activated sludge from the treated effluent, the self-immobilization of sludge is an attractive option because of its prompting solid-liquid separation in the clarifier. It has been recently reported that the biomass in the sequencing batch reactor (SBR) produces settling granules, which facilitates good solid-liquid separation and the accumulation of high amounts of activated sludge (Morgenroth et al., 1997; Beun et al., 1999; Etterer and Wilderer, 2001).
As compared with conventional activated sludge flocs, the advantages of granular activated sludge are compact and strong in structure (Etterer and Wilderer, 2001). It also has good settle ability and high capacity for biomass retention, and is able to withstand high organic loading rates (Morgenroth et al., 1997). With molasses as the sole carbon source, round-shaped aerobic granules with an average diameter of 0.6 mm started to appear in an SBR after around 40 days of operation. In an aerobic SBR fed with sodium acetate, granules with a diameter of 0.3-0.5 mm were formed after one month of operation (Peng et al., 1999). Large-sized aerobic granules with an average diameter of 3.3 mm were produced in an SBR fed with ethanol and operated at a short hydraulic retention time of 6.75 h and influent chemical oxygen demand (COD) of 830 mg/l. A relative high shear was found favorable for granulation (Beun et al., 1999). Aerobic granules could be formed within 3 weeks in two SBRs fed respectively with glucose and acetate (Tay et al., 2002).
However, so far, information about the physicochemical characteristics of aerobic granules grown on different carbon sources, especially protein, is still sparse. Therefore, the main objective of this work was to explore the granulation process and to compare the physicochemical properties of granules cultivated respectively with sucrose- and protein-rich wastewaters.
Materials and Methods
Experimental Set-Up and Operation
Two SBRs were used in this study. The SBR receiving the synthetic sucrose-rich wastewater was designated as RC, while another treating the soybean-processing wastewater was designated as RS. Each reactor had a working volume of 2.2 liters with an internal diameter of 6.0 cm and a height of 90 cm (Fig. 1). Air was introduced through an air diffuser by an air pump at the bottom of the reactor at an air velocity of 2.2 c d s . The airflow rate was control by a gas-flow controller. The temperature of the reactor was maintained at 25°C.
Both reactors were operated in a fill-draw mode. R,-was operated at a loading rate of 3.75 kgCOD/(m3.d) with a hydraulic retention time of 480 min and influent COD of 1250 mg/l. RC had successive cycles of 240 min each. One cycle consisted of 10 min of influent addition, 220 min of aeration, 5 min of settling, and 5 min of effluent withdrawal. For RS, it was operated at a loading rate of 6.00 kgCOD/(m3.d) with a hydraulic retention time of 240 min and influent COD of 2100 mg/l. It had 5 min of influent filling, 220 min of aeration, 5 min of settling and 10 min of effluent withdrawal. For both reactors, effluent was withdrawn from the port at 50 cm from the reactor bottom with a volumetric ratio of 50%.
Activated sludge from a local municipal wastewater treatment plant was used as inoculurn. The inoculum was seeded to each reactor, resulting in initial mixed liquor volatile suspended solids (MLVSS) of 3000 mg/l in RC and 3400 mg/l in RS.
Wastewater
The composition of the synthetic wastewater was as follows (in mgA): sucrose, 830; peptone, 250; beef extract, 160; NH4C1, 125; K2HPO4, 30; CaCl2, 20; MgS04, 15; FeSO47H2O, 15. The protein-COD accounted for 30% of total-COD. The trace element solution contained (in mg/l): H3BO3, 50; ZnCl2, 50; CuCl2, 30; MnSO4H2O, 50; (NH4)6Mo7O244H2O, 50; AlCl3, 50; CoCl26H2O, 50 and NiCl2, 50. The influent pH value was adjusted to 7.0 by the addition of NaHCO3 and H2SO4.
A protein-laden wastewater, obtained from a local soybean-processing plant, was used to feed RS. This wastewater had 2100 mg/l of COD, 547 mg/l of proteins, 422 mg/l of carbohydrates and 574 mg/l of total nitrogen. The soluble COD of the wastewater was 1650 mg/l. Since the wastewater contained sufficient amounts of nitrogen, only phosphorus as Na2HPO4 was added to ensure the ratio of COD to P to be 100: 1. The trace element solution was also dosed.
Analytical Methods
Microbial observation was conducted by using anoptical microscope (Olympus CX41). The granular activated sludge size was measured by an image analysis system (Image-pro Express 4.0, Media Cybernetics) with an Olympus CX31 microscope and a digital camera (Olympus (25050 Zoom).
The extracellular polymeric substances (EPS) of sludge were extracted by using EDTA method (Duncan-Hewitt et al., 1989). The carbohydrate concentration in EPS was determined as glucose equivalent using the Dubois method (1956). Protein concentration was measured as bovine albumin equivalent using the Lowry method (1951). The hydrophobic nature of the activated sludge particles was determined by measuring contact angle by axisymmetric drop shape analysis following the method proposed by Duncan-Hewitt et al. (1989).
(Continues…)
Excerpted from "Aerobic Granular Sludge"
by .
Copyright © 2005 IWA Publishing.
Excerpted by permission of IWA Publishing.
All rights reserved. No part of this excerpt may be reproduced or reprinted without permission in writing from the publisher.
Excerpts are provided by Dial-A-Book Inc. solely for the personal use of visitors to this web site.
Table of Contents
Preface, vii,
The unity of biofilm structures M.C.M. van Loosdrecht, M.K. de Kreuk and J.J. Heijnen, 1,
Comparison of the granulation of activated sludge grown on carbohydrate- and protein-rich wastewaters Han-Qing Yu, Yu-Ming Zheng and Kui-Zu Su, 7,
Characteristics and applicability of nitrifying granules produced in an aerobic upflow fluidized bed reactor S. Tsuneda, Y. Ejiri, M. Ogiwara, T. Nagano and A. Hirata, 15,
Aerobic granulation during the start up of a periodic biofilter C. Di Iaconi, R. Ramadori and A. Lopez, 25,
Study on the stability of aerobic granules in a SBAR-effect of superficial upflow air velocity and carbon source F. Wang, Y.-H. Liu, F.-L. Yang, XW. Zhang and H.-M. Zhang, 35,
Microstructural optimization of wastewater treatment by aerobic granular sludge V. Ivanov, J.-H. Tay, Q.-S. Liu, X.-H. Wang, Z.-W. Wang, B.A.M. Maszenan, S. Yi, W.-Q. Zhuang, Y.-Q. Liu, S. Pan and S.T.-L. Tay, 43,
Population dynamics during aerobic granule formation: lessons from denaturing gradient gel electrophoresis B.S. McSwain, R.L. Irvine and P.A. Wilderer, 53,
Nitrifying granular sludge in a sequencing batch reactor A. Mosquera-Corral, J.R. Vázquez-Padín, B. Arrojo, J.L. Campos and R. Méndez, 63,
Simultaneous phosphorus and nitrogen removal by aerobic granular sludge in single SBR system X.M. Li, G.J. Yang, Q. Yang, G.M. Zeng, D.X. Liao, M.F. Hu and Y.M. Wu, 71,
Bio-P removal profile of aerobic granular activated sludge from an anaerobic/aerobic SBR system J. Zhu, C. Liu and P.A. Wilderer, 79,
Developing aerobic phenol-degrading granules from acetate-fed granules S.T.-L. Tay, B.Y.-P. Moy, A.M. Maszenan and J.-H. Tay, 87,
Treatment of food industry effluents in a granular sludge SBR N. Schwarzenbeck and P.A. Wilderer, 95,
Modelling nutrient removal of an aerobic granular sludge lab-scale SBR using ASM3 M. Lübken, N. Schwarzenbeck, M. Wiehern, P.A. Wilderer, 103,
Aerobic granular sludge - from idea to pilot plant M.K. de Kreuk, L.M.M. de Bruin and M.C.M. van Loosdrecht, 111,
A comparative study of aerobic granulation in pilot- and laboratory-scale SBRs J.-H. Tay, Q.-S. Liu, Y. Liu, K.-Y. Show, V. Ivanov and S.T.-L. Tay, 125,
Promising results pilot research aerobic granular sludge technology at WWTP Ede L.M.M. de Bruin, H.F. van der Roest, M.K. de Kreuk, M.C.M. van Loosdrecht, 135,
Risks of aerobic granular sludge technology; ethical and methodological aspects I.R. van de Poel, S.D. Zwart, M. Brümsen, H.G.J. van Mil, 143,
Discussion Outcomes M. K. de Kreuk, B.S. McSwain, S. Bathe, S.T.L. Tay, N. Schwarzenbeck and P.A. Wilderer, 155,