Analysis of Evolutionary Processes: The Adaptive Dynamics Approach and Its Applications available in Hardcover
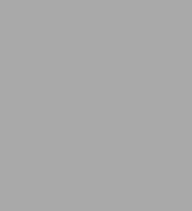
Analysis of Evolutionary Processes: The Adaptive Dynamics Approach and Its Applications
- ISBN-10:
- 0691120064
- ISBN-13:
- 9780691120065
- Pub. Date:
- 03/02/2008
- Publisher:
- Princeton University Press
- ISBN-10:
- 0691120064
- ISBN-13:
- 9780691120065
- Pub. Date:
- 03/02/2008
- Publisher:
- Princeton University Press
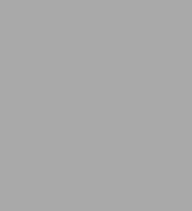
Analysis of Evolutionary Processes: The Adaptive Dynamics Approach and Its Applications
Hardcover
Buy New
$130.00Overview
After introducing the basics of evolutionary processes and classifying available modeling approaches, Dercole and Rinaldi give a detailed presentation of the derivation of the AD canonical equation, an ordinary differential equation that focuses on evolutionary processes driven by rare and small innovations. The authors then look at important features of evolutionary dynamics as viewed through the lens of AD. They present their discovery of the first chaotic evolutionary attractor, which calls into question the common view that coevolution produces exquisitely harmonious adaptations between species. And, opening up potential new lines of research by providing the first application of AD to economics, they show how AD can explain the emergence of technological variety.
Analysis of Evolutionary Processes will interest anyone looking for a self-contained treatment of AD for self-study or teaching, including graduate students and researchers in mathematical and theoretical biology, applied mathematics, and theoretical economics.
Product Details
ISBN-13: | 9780691120065 |
---|---|
Publisher: | Princeton University Press |
Publication date: | 03/02/2008 |
Series: | Princeton Series in Theoretical and Computational Biology , #3 |
Pages: | 360 |
Product dimensions: | 6.00(w) x 9.25(h) x (d) |
About the Author
Read an Excerpt
Analysis of Evolutionary Processes The Adaptive Dynamics Approach and Its Applications
By Fabio Dercole Sergio Rinaldi Princeton University Press
Copyright © 2008 Princeton University Press
All right reserved.
ISBN: 978-0-691-12006-5
Chapter One Introduction to Evolutionary Processes
In this chapter we introduce the basic elements and the empirical evidence of evolutionary processes. Since the groundbreaking work The Origin of Species by Charles Darwin (1859), a great deal of effort has been dedicated to the subject (see, e.g., Fisher, 1930; Haldane, 1932; Dobzanski, 1937; Mayr, 1942, 1963, 1982; Wright, 1969; Dawkins, 1976, 1982, 1986; Cavalli-Sforza and Feldman, 1981; Maynard Smith, 1989, 1993; Maynard Smith and Szathmary, 1995, just to mention a few masterpieces). Our discussion on the origin of evolutionary theory is mainly taken from the introduction by Ernst Mayr (2001) to the seventeenth printing of Darwin's famous book, and from Dieckmann (1994, Chapter 1), Schrage (1995), Rizzoli-Larousse (2003), and the web pages of the University of California Museum of Paleontology. Throughout the exposition we emphasize that, even though the major scientists who developed evolutionary theory were stimulated by the study of nature, their ideas not only apply to the biological realm, but also capture many phenomena of self-organization encountered in social sciences, economics, and engineering.
1.1 ORIGINS OF EVOLUTIONARY THEORY
The idea that living organisms havebeen diversifying themselves through time, starting from a common origin, goes back to the Greek naturalistic philosophy. Among the precursors of evolutionary theory, as we define it today, we can mention Anaximander of Miletus (610-546 BC), Empedocles of Acragas (495-435 BC), and later some clergymen, such as Saint Augustine (354-430). The evolutionary conceptions of Greek philosophy were known during the Renaissance. However, no further contribution arose until the eighteenth century, when European scholars still believed that the universe was created in essentially its present and final state. During the eighteenth century, the work of intellectuals known as "encyclopedists" spread the Illuminism doctrine and, in particular, the results of pioneering research in systematic biology, aimed at hierarchically classifying organisms into groups that successively share more and more visible structural characteristics. Their work brought a better understanding of the concept of species and highlighted fundamental similarities between widely disparate organisms. Such similarities were in contrast with the hypothesis of creation in final state and prepared the ground for evolutionary theory. A considerable contribution came from Georges-Louis Leclerc Buffon (1707-1788), author of a compendium of biological history, and from Erasmus Darwin (1731-1802), Charles' grandfather, who first discussed the conjecture that life could have evolved from a common ancestor and posed the question of how a species could evolve into another. The first explicit evolutionary theory was formulated by Jean Baptiste Lamarck (1744-1829), disciple of Buffon, who introduced the notion of inheritance. The "Lamarckian" hypothesis, that simple life forms continually come into existence from dead matter and continually become more complex, was strongly criticized by most naturalists of the time. In particular, one of the most active antievolutionists, Georges Cuvier (1769-1832), paradoxically provided evidence to the evolutionary hypothesis with his research in systematic biology, comparative anatomy, and paleontology.
At this point Charles Darwin (1809-1882) and Alfred Russel Wallace (1823-1913) formulated the evolutionary theory that we still accept today. In their papers published in the same issue of the Journal of the Proceedings of the Linnean Society (Darwin, 1858; Wallace, 1858, often cited as a single paper with the title "On the Tendency of Species to form Varieties; and on the Perpetuation of Varieties and Species by Natural Means of Selection") they presented their theory of evolution by natural selection, arguing that
there is individual variation in innumerable characteristics of populations, some of which may affect the individual ability to survive and reproduce;
there is likely to be a hereditary component to much of this variation, but evolutionary change ultimately relies on the appearance of new variant forms of organisms, called mutants;
generation by generation there is a natural selection of the characteristics associated with greater survival and reproductive success, whose frequencies in the populations increase over time;
the cumulative effects of mutations and natural selection, over a long period of time, alter the characteristics of species from those of their ancestors;
all living organisms have descended with modifications from a common ancestor, thus developing hierarchical patterns of similarities.
Darwin and Wallace combined empirical observations with theoretical insights gained from Malthus' (1798) work on competition and population growth. They had a precise idea of natural selection and realized the need of mutations. However, they were not aware of the laws of heredity, discovered seven years later by Gregor Mendel (1822-1884), who realized the discrete nature of heredity determinants, which we now call genes (Mendel, 1865). Darwin actually introduced the concept of natural selection and deserves, more than anyone else, the credit for having started, and firmly supported, the scientific and philosophical revolution from the dogma of creation and constancy of species to evolutionary theory.
The decisive event in Darwin's life was the five-year period spent as a naturalist on the vessel Her Majesty's Ship Beagle (from December 1831 to October 1836), in which he surveyed the coast of South America and the off-lying islands, collecting invaluable observations on the tropical forests of Brazil, on fossils in the Pampas of Argentina, on the geology of the Andes, and on the animal life of the Galapagos Islands (Darwin, 1839). After the return of the Beagle, Darwin spent most of his time in the analysis and interpretation of his findings and became later more acknowledged than Wallace, thanks to his famous book The Origin of Species (1859).
A third important scientist in the development of evolutionary theory, though by far less acknowledged than Darwin and Wallace, is Patrick Matthew (1790-1874). In a letter to Charles Lyell (April 10, 1860) Darwin says: "In last Saturday Gardeners' Chronicle, a Mr. Patrick Matthew publishes long extract from his work on Naval Timber & Arboriculture published in 1831, in which he briefly but completely anticipates the theory of Natural Selection. - I have ordered the book, as some few passages are rather obscure but it is, certainly, I think, a complete but not developed anticipation." Matthew's evolutionary insights lie buried in an appendix of a book he wrote on raising trees of optimal quality for the Royal Navy (Matthew, 1831). In that appendix Matthew expressed his theory based on how tree species might vary in form and how artificial selection might improve cultivated trees.
Let us now listen directly to Darwin, Wallace, and Matthew. "The affinities of all the beings of the same class have sometimes been represented by a great tree. I believe this simile largely speaks the truth. The green and budding twigs may represent existing species; and those produced during each former year may represent the long succession of extinct species ... The limbs divided into great branches, and these into lesser and lesser branches, were themselves once, when the tree was small, budding twigs; and this connexion of the former and present buds by ramifying branches may well represent the classification of all extinct and living species in groups subordinate to groups ... From the first growth of the tree, many a limb and branch has decayed and dropped off, and these lost branches of various sizes may represent those whole orders, families, and genera which have now no living representatives, and which are known to us only from having been found in a fossil state ... As buds give rise by growth to fresh buds, and these, if vigorous, branch out and overtop on all a feebler branch, so by generation I believe it has been with the Tree of Life, which fills with its dead and broken branches the crust of the earth, and covers the surface with its ever branching and beautiful ramifications" (Darwin, 1859).
"We have also here an acting cause to account for that balance so often observed in Nature - a deficiency in one set of organs always being compensated by an increased development of some others - powerful wings accompanying weak feet, or great velocity making up for the absence of defensive weapons; for it has been shown that all varieties in which an unbalanced deficiency occurred could not long continue their existence. The action of this principle is exactly like that of the centrifugal governor of the steam engine, which checks and corrects any irregularities almost before they become evident; and in like manner no unbalanced deficiency in the animal kingdom can ever reach any conspicuous magnitude, because it would make itself felt at the very first step, by rendering existence difficult and extinction almost sure soon to follow" (Wallace, 1858).
"As Nature, in all her modifications of life, has a power of increase far beyond what is needed to supply the place of what falls by Time's decay, those individuals who possess not the requisite strength, swiftness, hardihood, or cunning, fall prematurely without reproducing - either a prey to their natural devourers, or sinking under disease, generally induced by want of nourishment, their place being occupied by the more perfect of their own kind, who are pressing on the means of subsistence. . . a law universal in Nature, tending to render every reproductive being as the best possibly suited to its condition ... There is more beauty and unity of design in this continual balancing of life to circumstance, and greater conformity to those dispositions of Nature which are manifest to us, than in total destruction and new creation. It is improbable that much of this diversification is owing to commixture of species nearly allied, all change by this appears very limited, and confined within the bounds of what is called species; the progeny of the same parents, under great differences of circumstance, might, in several generations, even become distinct species, incapable of co-reproduction" (Matthew, 1831).
After more than a century since the publication of Darwin's famous book, we can say that the impact it had on man's concept of himself and his activities has been dramatic and has gone far beyond biology. Reduced to the essential, evolutionary change can be described as a two-step process: the first step consists of innovation, namely the production of variations, while the second is ruled by competition and leads to the selection of the best-performing variants. This abstract paradigm can be applied to many processes of self-organization that drive the evolution of complex natural and artificial systems, composed of several interacting agents, or units, each characterized by individual traits that are transmitted, with possible modifications, to agents or units of next generation, and naturally or artificially selected by their effectiveness or by optimization criteria. In other words, after the Darwinian "revolution," networks of socio-cultural relationships, the global economy, and the design of several industrial processes can be interpreted and studied as evolutionary processes.
In particular, Richard Dawkins reformulated the paradigm of evolution independently of genetic inheritance to explain the evolution of culture. In his books The Selfish Gene (1976) and The Extended Phenotype (1982) he argued that cultures, namely the clouds of ideas, behavioral traits, and artifacts developed and produced by animal and human populations, compete, cooperate, mutate, and are transmitted as well as genetic traits. Thus, the constitutive elements of cultures do evolve, and actually coevolve with genetic traits in a whole biological-sociocultural evolutionary process. Dawkins introduced the concept of a replicator as the minimum natural or artificial unit evolvable through a mutation-selection (or better innovation-competition) process. Replicators are characterized by four fundamental properties:
replication: units generate or are replaced by new units;
transmission: units are characterized by distinctive features that are passed to the units of next generation;
innovation: transmission is not a perfect copy but allows for variations;
competition: survival and effectiveness of units are regulated by the characteristic features of interacting units.
Dawkins' idea of a replicator was elaborated on just a few years after Francis Crick and James Watson's (1953) discovery of the double helix molecular structure of the deoxyribonucleic acid (DNA). Although this idea might simply seem an abstract summary of Darwin and Wallace's evolutionary theory, its conceptual impact is enormous because it offers a paradigm of evolution that can be scaled from DNA to macroscopic natural and artificial systems. In fact, even if Dawkins' work is focused on animal and, in particular, human biological and cultural coevolution, the concept of replicator is fully independent of biology and can in principle be applied to describe any innovation-competition process. However, for tradition, simplicity, and uniformity, we will mainly refer throughout this chapter to biological evolution, pointing out here and there, and, in particular, in Section 1.9, the analogy with the evolution of social and economic systems. Later, in Chapter 4, we will interpret and analyze the process of technological change as an evolutionary process, where replicators are commercial products competing in a market, which replicate in production and transmit, with possible innovations, technological characteristics to new generations of products. Through a stylized model we will show that the evolutionary interpretation of technological change supports the emergence of technological variety from a single ancestral technology.
In the next three sections we introduce the basic biological elements of evolutionary processes, namely the structure of the genetic material and the laws of heredity, underpinning the way of being and reproducing of all living organisms, the appearance of mutations, ultimate source of organisms variability, and the mechanisms of selection of successful mutants. Among the innumerable options, we closely follow Charlesworth and Charlesworth (2003), a lucid and concise introduction to evolutionary theory, presenting some of their contributions in a form appropriate for this book. Sections 1.5-1.8 describe the evolutionary patterns we might expect to emerge as long-term consequences of evolutionary processes, while the last section shows many examples of such patterns in biological as well as nonbiological contexts.
1.2 GENOTYPES AND PHENOTYPES
Starting with Mendel's (1865) work and passing through the achievements of modern molecular biology, we now know that the similarities between living organisms are not confined to visible structural characteristics, but are profound and extend to the smallest microscopic scale. All living organisms other than viruses (which are on the borderline of life) are composed of a single unit or an assembly of essentially similar units, the cells. In so-called eukaryote organisms, which include all multicellular species (animals, plants, and fungi) as well as some unicellular species, cells are delimited by a membrane and contain the cytoplasm, a gel with floating subcellular structures, and the nucleus, which carries the genetic material. The remaining unicellular organisms, called prokaryotes and including bacteria and similar organisms called archaea, are simpler cells in which the genetic material is floating in the gel with no subcellular structures and nucleus. Viruses are parasites that reproduce inside the cells of other organisms and consist of a chemical coat surrounding the genetic material.
Cells are miniaturized factories that produce the chemicals needed by the organism, generate energy from food, and provide body structures. Most chemical products of a cell, as well as the machinery employed for the production of chemicals and energy, are proteins. Some proteins are enzymes that take a chemical and perform a task on it, like breaking molecules in food into smaller pieces that can be assimilated by cells. Some other proteins have storage or transport functions, like binding to iron and storing it in the liver, or to oxygen and carrying it through the blood. Some others are communication proteins, like hormones, which circulate in the blood and control many functions, or signaling proteins, located on the cell surface and responsible of communications with other cells. Finally, there are structural proteins, e.g., those forming skin and bones. Proteins are large molecules composed of a chain of amino acids typically arranged in a suitable spatial configuration. There are only twenty different amino acids across the entire range of living organisms. This points out how similar all organisms are at a microscopic scale and indirectly supports the evolutionary hypothesis of a common ancestor.
(Continues...)
Excerpted from Analysis of Evolutionary Processes by Fabio Dercole Sergio Rinaldi
Copyright © 2008 by Princeton University Press. Excerpted by permission.
All rights reserved. No part of this excerpt may be reproduced or reprinted without permission in writing from the publisher.
Excerpts are provided by Dial-A-Book Inc. solely for the personal use of visitors to this web site.
Table of Contents
Preface xi
Chapter 1. Introduction to Evolutionary Processes 1
1.1 Origins of Evolutionary Theory 1
1.2 Genotypes and Phenotypes 5
1.3 Mutations 9
1.4 Selection 10
1.5 Evolution 13
1.6 The Red Queen Hypothesis 16
1.7 The Emergence of Diversity 17
1.8 Evolutionary Extinction 21
1.9 Examples 24
Chapter 2. Modeling Approaches 43
2.1 Overview 43
2.2 Population Genetics 47
2.3 Individual-based Evolutionary Models 53
2.4 Quantitative Genetics 55
2.5 Evolutionary Game Theory 59
2.6 Replicator Dynamics 62
2.7 Fitness Landscapes 64
2.8 Adaptive Dynamics 67
2.9 A Comparative Analysis 70
Chapter 3. The Canonical Equation of Adaptive Dynamics 74
3.1 The Evolving Community 74
3.2 The Resident-Mutant Model 76
3.3 The Example of Resource-Consumer Communities 79
3.4 Does Invasion Imply Substitution? 83
3.5 The AD Canonical Equation 88
3.6 Evolutionary State Portraits 95
3.7 Evolutionary Branching 99
3.8 The Role of Bifurcation Analysis 110
3.9 What Should We Expect from the AD Canonical Equation 116
Chapter 4. Evolutionary Branching and the Origin of Diversity 119
4.1 Introduction 119
4.2 A Market Model and Its AD Canonical Equation 121
4.3 A Simple Example of Technological Branching 129
4.4 Discussion and Conclusions 135
Chapter 5. Multiple Attractors and Cyclic Evolutionary Regimes 138
5.1 Introduction 138
5.2 A Model of Resource-Consumer Coevolution 139
5.3 The Catalog of Evolutionary Scenarios 144
5.4 Discussion and Conclusions 151
Chapter 6. Catastrophes of Evolutionary Regimes 153
6.1 Introduction 153
6.2 A Model for the Evolution of Cooperation 154
6.3 Catastrophic Disappearance of Evolutionary Attractors 159
6.4 Evolutionary Branching and the Origin of Cheaters 166
6.5 Discussion and Conclusions 169
Chapter 7. Branching-Extinction Evolutionary Cycles 172
7.1 Introduction 172
7.2 A Model of Cannibalistic Demographic Interactions 174
7.3 Coevolution of Dwarfs and Giants 177
7.4 The Branching-Extinction Evolutionary Cycle 182
7.5 Discussion and Conclusions 183
Chapter 8. Demographic Bistability and Evolutionary Reversals 186
8.1 Introduction 186
8.2 Biological Background 188
8.3 Asymmetric Competition and the Occurrence of Evolutionary Reversals 189
8.4 Slow-Fast Approximation of the AD Canonical Equation 195
8.5 Discussion and Conclusions 200
Chapter 9. Slow-Fast Populations Dynamics and Evolutionary Ridges 204
9.1 Introduction 204
9.2 Biological Background 207
9.3 The AD Canonical Equation for General Demographic Attractors 209
9.4 Evolutionary Sliding and Pseudo-equilibria 221
9.5 Results and Discussion 224
9.6 Concluding Remarks 229
Chapter 10. The First Example of Evolutionary Chaos 231
10.1 Introduction 231
10.2 A Tritrophic Food Chain Model and Its AD Canonical Equation 233
10.3 The Chaotic Evolutionary Attractor 235
10.4 Feigenbaum Cascade of Period-doubling Bifurcations 238
10.5 Discussion and Conclusions 241
Appendix A. Second-order Dynamical Systems and Their Bifurcations 243
A.1 Dynamical Systems and State Portraits 243
A.2 Structural Stability 248
A.3 Bifurcations as Collisions 250
A.4 Local Bifurcations 252
A.5 Global Bifurcations 259
A.6 Catastrophes, Hysteresis, and Cusp 261
A.7 Extinction Bifurcations 265
A.8 Numerical Methods and Software Packages 267
Appendix B. The Invasion Implies Substitution Theorem 272
Appendix C. The Probability of Escaping Accidental Extinction 277
Appendix D. The Branching Conditions 281
Bibliography 287
Index 325
What People are Saying About This
The first comprehensive textbook on the methods and applications of adaptive dynamics, Analysis of Evolutionary Processes is very timely indeed. It will be of great interest not only to researchers already using AD but also to those who want to apply it but are not yet familiar with the methods. Dercole and Rinaldi's book is well written, self-contained, and suitable for self-study and teaching in applied mathematics and mathematical biology on the graduate and advanced undergraduate levels.
Stefan A. H. Geritz, University of Helsinki
"The first comprehensive textbook on the methods and applications of adaptive dynamics, Analysis of Evolutionary Processes is very timely indeed. It will be of great interest not only to researchers already using AD but also to those who want to apply it but are not yet familiar with the methods. Dercole and Rinaldi's book is well written, self-contained, and suitable for self-study and teaching in applied mathematics and mathematical biology on the graduate and advanced undergraduate levels."—Stefan A. H. Geritz, University of Helsinki"This is a major achievement—a self-contained presentation of the adaptive dynamics approach, of its role within evolutionary theory, and of the kind of evolutionary dynamics that can be predicted. I believe it will become a standard text for researchers and students in evolutionary dynamics. To my knowledge there is no other book that presents the theory of AD, places it in a proper biological context, and develops it with an approach that is mathematically sound but not overwhelming."—Andrea Pugliese, University of Trento, Italy
The first comprehensive textbook on the methods and applications of adaptive dynamics, Analysis of Evolutionary Processes is very timely indeed. It will be of great interest not only to researchers already using AD but also to those who want to apply it but are not yet familiar with the methods. Dercole and Rinaldi's book is well written, self-contained, and suitable for self-study and teaching in applied mathematics and mathematical biology on the graduate and advanced undergraduate levels.
Stefan A. H. Geritz, University of Helsinki
This is a major achievementa self-contained presentation of the adaptive dynamics approach, of its role within evolutionary theory, and of the kind of evolutionary dynamics that can be predicted. I believe it will become a standard text for researchers and students in evolutionary dynamics. To my knowledge there is no other book that presents the theory of AD, places it in a proper biological context, and develops it with an approach that is mathematically sound but not overwhelming.
Andrea Pugliese, University of Trento, Italy