This volume explores animal body size from a macroecological perspective, examining species, populations, and other large groups of animals in order to uncover the patterns and causal mechanisms of body size throughout time and across the globe. The chapters represent diverse scientific perspectives and are divided into two sections. The first includes chapters on insects, snails, birds, bats, and terrestrial mammals and discusses the body size patterns of these various organisms. The second examines some of the factors behind, and consequences of, body size patterns and includes chapters on community assembly, body mass distribution, life history, and the influence of flight on body size.
This volume explores animal body size from a macroecological perspective, examining species, populations, and other large groups of animals in order to uncover the patterns and causal mechanisms of body size throughout time and across the globe. The chapters represent diverse scientific perspectives and are divided into two sections. The first includes chapters on insects, snails, birds, bats, and terrestrial mammals and discusses the body size patterns of these various organisms. The second examines some of the factors behind, and consequences of, body size patterns and includes chapters on community assembly, body mass distribution, life history, and the influence of flight on body size.
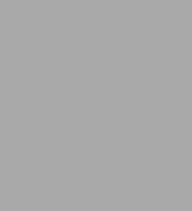
Animal Body Size: Linking Pattern and Process across Space, Time, and Taxonomic Group
280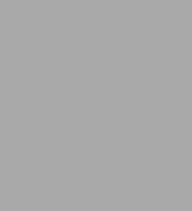
Animal Body Size: Linking Pattern and Process across Space, Time, and Taxonomic Group
280Hardcover
-
PICK UP IN STORECheck Availability at Nearby Stores
Available within 2 business hours
Related collections and offers
Overview
This volume explores animal body size from a macroecological perspective, examining species, populations, and other large groups of animals in order to uncover the patterns and causal mechanisms of body size throughout time and across the globe. The chapters represent diverse scientific perspectives and are divided into two sections. The first includes chapters on insects, snails, birds, bats, and terrestrial mammals and discusses the body size patterns of these various organisms. The second examines some of the factors behind, and consequences of, body size patterns and includes chapters on community assembly, body mass distribution, life history, and the influence of flight on body size.
Product Details
ISBN-13: | 9780226012148 |
---|---|
Publisher: | University of Chicago Press |
Publication date: | 08/09/2013 |
Pages: | 280 |
Product dimensions: | 6.00(w) x 9.10(h) x 0.80(d) |
About the Author
Read an Excerpt
Animal Body Size
Linking Pattern and Process across Space, Time, and Taxonomic Group
By FELISA A. SMITH, S. KATHLEEN LYONS
THE UNIVERSITY OF CHICAGO PRESS
Copyright © 2013 The University of ChicagoAll rights reserved.
ISBN: 978-0-226-01214-8
CHAPTER 1
Macroecological Patterns in Insect Body Size
Kevin J. Gaston and Steven L. Chown
Introduction
Imagine a continuum representing the level of understanding of macroecological patterns in body size. Toward one extreme lie groups like birds and mammals, for which many of these patterns have been well documented, their mechanisms have been extensively explored (albeit not necessarily resolved), and the level of understanding is being driven vigorously forward. Toward the other lie the insects, for which many patterns are poorly documented, the mechanisms remain little explored, and the level of understanding is often rather limited. The insects are found in this position in major part for two reasons. The first is the extraordinarily large numbers of species. Best estimates are that there are 4–5 million extant species of insects, of which, depending in marked degree on the extent of synonymy, perhaps 0.75–1 million have been formally taxonomically described (previous much higher estimates of overall species richness have largely been discounted—Gaston and Hudson 1994; Gauld and Gaston 1995; May 2000; Ødegaard et al. 2000; Dolphin and Quicke 2001; Novotný et al. 2002; Chapman 2009). The second reason is that the sampling of this diversity has been extremely heterogeneous in space, with, for example, the geographic distribution of those species that have been taxonomically described not conforming with predicted patterns of actual richness (Gaston 1994). Faced with such issues, the best that can usually be done is to tackle the macroecological patterns in particular, less speciose higher taxa, such as tribes or families, and often then only for limited regions of the world. Nonetheless, given that insects are likely to constitute 60% or more of all extant eukaryote species (and a substantial proportion of all the eukaryotic species that have ever existed), any claim to a solid general understanding of the macroecological patterns of body size requires more than an acknowledgment that studies of these issues are difficult for insects (Diniz-Filho et al. 2010). This is particularly so given that examples from the insects are widely employed to support general arguments about the determinants of macroecological patterns in body size, such as the limitations on size posed by structural constraints of body plans, and the need for a paleontological perspective on present-day trends.
In this chapter, we provide an overview of the current understanding of the form of macroecological patterns in insect body size, with particular emphasis on global patterns, patterns through time, and patterns through space. Throughout, we concentrate foremost on patterns at large geographic scales, rather than those in the body sizes of particular local assemblages, although the latter have received substantially more attention (e.g., Janzen 1973; Morse et al. 1988; Basset and Kitching 1991; Basset 1997; Siemann et al. 1999b; Hodkinson and Casson 2000; Krüger and McGavin 2000; Ulrich 2004). The proximate physiological and cellular mechanisms that regulate body size are also not dealt with here. They are the subject of a large literature (e.g., Nijhout 2003; Davidowitz et al. 2005; Edgar 2006; Nijhout et al. 2006; Mirth and Riddiford 2007; Whitman and Ananthakrishnan 2009; see also Chown and Gaston 2010), and understanding in the area is developing rapidly (e.g., Nijhout et al. 2010; Stillwell and Davidowitz 2010a, 2010b; Callier and Nijhout 2011). Nonetheless, we touch on these mechanisms where relevant in a macroecological context.
Global Patterns
The Smallest and the Largest
The variation in body size exhibited by extant insects is marked. At the level of the individual, the increase in size from egg to adult can be as much as 43,000% (Klok and Chown 1999), and may be larger (see also Whitman 2008). Typically, this variation has substantial implications for the resources used by growing individuals, often entailing fundamentally different approaches to acquisition at different life stages (e.g., Gaston et al. 1991), with the most extreme changes being represented by alterations both in size and habitat in aquatic insects with aerial adult stages. Ontogenetic changes in insect body size also alter the kinds of predators that feed on them. In anomalous emperor moth Imbrasia belina caterpillars (or mopane worms), the early instars are eaten by small predators such as insects and gleaning birds, the later instars by a variety of insectivorous birds, and the last instar by reptiles, mammalian carnivores, and humans (Gaston et al. 1997).
Variation in size among individuals of a given species at a given life stage—constrained intraspecific variation (sensu Spicer and Gaston 1999)—can also be substantial. At least some of this variation is a consequence of food availability to juvenile stages (Emlen 1997b; Emlen and Nijhout 2000; Peat et al. 2005; Whitman 2008). In turn, size variation may have substantial effects on fecundity (Honek 1993), resource allocation trade-offs, and mating strategies. The latter are particularly well known in beetles (Emlen and Nijhout 2000) but are also found in exopterygotes (e.g., Tomkins and Brown 2004). In many social species, size variation is determined by caste membership and variation within a given caste (Oster and Wilson 1978; Fraser et al. 2000; Hughes et al. 2003). More extreme cases include leaf-cutter ants Atta spp. and army ants Eciton hamatum, where workers range from 0.0025 to 0.0206 g and from 0.0017 to 0.027 g, respectively (Feener et al. 1988; Roces and Lighton 1995). Considerable intraspecific plasticity means that variation in the size of individual species can perhaps more closely approach variation in the average sizes of different species within some taxonomic groups of insects than is the case for taxa of many other kinds of organisms. In other words, the extent to which, say, the mean or median size of individuals of a given life stage adequately characterizes the body size of a species for the purposes of comparative analyses is less secure for insects than it is for many other taxa. Using average sizes instead of the size of the individual of the given species of interest can result in substantially different, and perhaps incorrect, interpretations of the relationships between body size and ecosystem properties (Cohen et al. 2005).
Across the insects as a whole, the smallest species has been argued to be the mymarid egg-parasitic wasp Dicopomorpha echmepterygis, males of which are wingless and measure as little as 139 µm, with females being approximately 40% larger (Gahlhoff 1998). Given that this species was not described until 1997, it is not unlikely that there are yet smaller species still to be found. Although there is much debate, five beetle species have been argued to contend the claim to be the largest insect in terms of measurable bulk: the cerambycid Titanus giganteus (167 mm), the elephant beetles Megasoma elephas (137 mm) and M. actaeon (135 mm), and the goliath beetles Goliathus goliatus and G. regius (110 mm) (Williams 2001). This gives a range of body lengths for adult insects of three orders of magnitude. Across the beetles alone, species body lengths may vary to a similar extent, with the feather-winged beetles (Ptiliidae) being as small as 250 µm (Gahlhoff 1998).
The reliance here on length, rather than mass, as a measure of insect body size reflects a general practical constraint. Collection and storage methods for insects often limit opportunities for the direct determination of fresh body masses, and most studies of macroecological patterns in insect body size have thus employed measures of "characteristic linear dimensions" (e.g., body length, forewing length, wingspan). Where body masses have been used, these are commonly derived from general allometric relationships with these dimensions (for such relationships see, e.g., Rogers et al. 1976; Gowing and Recher 1984; Sample et al. 1993; Benke et al. 1999; Kaspari and Weiser 1999; Mercer et al. 2001; Powell and Franks 2006), rather than direct measurement. These relationships typically have substantial variance about them, unless limited to taxonomically rather narrow groups of species, as might be obvious from the considerable variation in body form of higher taxa that have similar body masses (e.g., Bartholomew and Casey 1978). Nonetheless, direct body mass measurements for insects are not uncommon, and it is clear that there is substantial interspecific mass variation across the group. It is at least six orders of magnitude, ranging from the thrips Apterothrips apteris on Marion Island at 0.00004 g (Mercer et al. 2001) to the giant weta Deinacrida heteracantha, with females weighing up to 71 g (Whitman 2008). However, globally the range is probably seven orders of magnitude, given the small size of mymarid wasps. This range is greater than the approximately four orders of magnitude spanned by birds (Blackburn and Gaston 1994), similar to that of mammals (Smith et al. 2004) but smaller than that of fish, which range from the likely milligram masses of Paedocypris progenetica (Kottelat et al. 2006) to the basking and whale sharks, which weigh several thousands of kilograms, so spanning eight or perhaps more orders of magnitude.
The implications of interspecific size variation for physiological and life history traits have been explored in a wide variety of studies. They include changes in chemical composition (Woods et al. 2003; Lease and Wolf 2011), biochemistry (Darveau et al. 2005b; Darveau et al. 2005a), structural features (Lease and Wolf 2010), thermal physiology (Willmer and Unwin 1981; Stevenson 1985), flight performance (Stone and Willmer 1989; Dudley 2000a; Darveau et al. 2005b), metabolic rate and gas exchange characteristics (Chown et al. 2007; White et al. 2007), locomotion speed, costs of transport, and ability to transit different landscapes (Kaspari and Weiser 1999; Chown and Nicolson 2004), preferred microclimates (Kaspari 1993), food intake rates (Reichle 1968), resource use (Kirk 1991; Novotný and Wilson 1997), host specificity (Wasserman and Mitter 1978; Loder et al. 1997; Novotný and Basset 1999), contest competitive ability (Heinrich and Bartholomew 1979), egg size (García-Barros and Munguira 1997), development time (Honek 1999), and intrinsic rates of increase (Gaston 1988a). In some cases, such as interspecific size variation in metabolic rate, development rate, and mortality, there is likely to be considerable feedback, so that body size is as much a function of these variables as they are of body size at the intraspecific level (see Kozlowski and Weiner 1997; Kozlowski and Gawelczyk 2002). In combination these effects will determine optimum body size and eventually in turn the interspecific relationships.
Conservatism of Body Size
Along with a number of life history traits, the body size of organisms tends in general to be highly phylogenetically conserved. Among the insects, this is also true. In physiological traits, much of the variance is partitioned at the family and genus levels (Chown et al. 2002). Using body masses from the same database used to examine variance partitioning in physiological traits (and including only those genera for which data were available for two or more species), taxonomic orders account for approximately 3% of the variation, families for 38%, genera for 39%, and the remaining variation is partitioned at the species level (Chown and Gaston 2010). Studies of individual orders in particular regions have also found substantial variation being partitioned among higher-level taxa (Loder 1997; Brändle et al. 2000). This partitioning makes intuitive sense, given that within orders such as the Orthoptera, Coleoptera, Hemiptera, Hymenoptera, and Lepidoptera, species take on a wide range of sizes (fig. 1.1), while within a given family size ranges are smaller. However, it also seems likely that as more mass data become available, the variance will be partitioned further toward the generic level, but perhaps more so for the Coleoptera than for any other major order. In the beetles, families tend to be speciose with substantial interspecific size variation (e.g., Carabidae, Curculionidae, Elateridae, Scarabaeidae), while genera tend to be more similar in size, as has long been remarked for carabid beetles (den Boer 1979).
Body Size Distributions
INTRASPECIFIC. The body size distributions of the individuals of particular species have been surprisingly poorly documented for insects, with the exception of the ants and other social Hymenoptera, where frequency distributions of some, usually linear, measure of size are often provided to help understand the causes, consequences, and evolution of the caste distribution function (Oster and Wilson 1978; Hughes et al. 2003; Yang et al. 2004; Schöning et al. 2005; Billick and Carter 2007). At present, although many studies do not highlight the fact that they contain such data, intraspecific body size frequency distributions are readily available for approximately sixty species, representing seven orders and twenty-one families, a tiny portion of insect diversity (Gouws et al. 2011). Nonetheless, several generalities emerge. Among the non social insects, intraspecific body size frequency distributions of both mass and linear measurements are either normally distributed or slightly right skewed. Bimodality seems relatively uncommon. Nonetheless, owing to allometry, substantial variation in the shape of the distributions can exist among linear measures depending on what trait is quantified. In consequence, care must be exercised in selecting the trait to be used for linear measurements and in reporting such distributions (Whitman 2008; Gouws et al. 2011). Among the social insects (mostly ants, but also a termite and wasp species) intraspecific body size frequency distributions appear typicaly to be right skewed or bimodal (Gouws et al. 2011). However, these authors echo Oster and Wilson's (1978) remarks that perhaps only 15% of ant genera show size polymorphism, and that most species are monomorphic. Thus, most intraspecific body size frequency distributions are probably approximately normal, with perhaps some tendency to show a positive skew. Despite these general patterns, the form of intraspecific body size frequency distributions (rather than their central tendency, which we discuss below) need not be static, and may change through space and time for several reasons (Waddington et al. 1986; Lounibos 1994; Evans 2000; Roulston and Cane 2000; Yang et al. 2004; Peat et al. 2005; Couvillon et al. 2010).
Although the frequency distributions presented by Gouws et al. (2011) and others do not immediately reveal sexual size dimorphism (SSD), males are usually smaller than females in insects (Teder and Tammaru 2005; Whitman 2008; Stillwell et al. 2010). This may be true of whole higher taxa, such as the extant Mantophasmatodea (Klass et al. 2003), where the difference is substantial. In Karoophasma biedouwensis males weigh 54.9 ± 8.3 mg, and females 128.6 ± 7.6 mg (Chown et al. 2006). The opposite pattern is not uncommon, however (Stillwell et al. 2010). Moreover, in a range of species there is substantial male size polymorphism (in polymorphic ants and some other social Hymenoptera it is the females that are variable; Emlen and Nijhout 2000). Intraspecific variation in SSD may well be a consequence of adaptive canalization of reproductive traits (Fairbairn 2005). At the interspecific level, SSD typically increases with size when males are the larger sex but declines with size when females are larger. Rensch's rule, as this pattern has come to be known (Fairbairn 1997; Stillwell et al. 2010), has been found in some cases at the intraspecific level. However, among-population SSD typically does not conform to the rule (Blanckenhorn et al. 2006), and environmental conditions affect the degree rather than direction of SSD, often as a consequence of sex-related differences in growth and instar number (Teder and Tammaru 2005). Where Rensch's rule is found, it seems likely that greater plasticity in males than in females (the differential plasticity hypothesis), rather than sexual selection, might account for variation in SSD among populations (Fairbairn 2005). Sex-related differences in plasticity may also be the mechanism underlying a geographic version of Rensch's rule (Blanckenhorn et al. 2006). In addition to variation in phenotypic plasticity, strong sex-specific selection on size associated with season length may be responsible for the pattern (Blanckenhorn et al. 2006). Variation in nutritional resources and moisture may also play a role (Stillwell et al. 2007). Geographic variation in the relative sizes of males and females, its implications for SSD, and the mechanisms underlying this variation require further investigation (see Stillwell et al. 2007 and Hu et al. 2010, for examples; and Stillwell et al. 2010, for review).
(Continues...)
Excerpted from Animal Body Size by FELISA A. SMITH, S. KATHLEEN LYONS. Copyright © 2013 The University of Chicago. Excerpted by permission of THE UNIVERSITY OF CHICAGO PRESS.
All rights reserved. No part of this excerpt may be reproduced or reprinted without permission in writing from the publisher.
Excerpts are provided by Dial-A-Book Inc. solely for the personal use of visitors to this web site.
Table of Contents
PrefaceAcknowledgments
Introduction: On Being the Right Size: The Importance of Size in Life History, Ecology and Evolution
Felisa A. Smith and S. Kathleen Lyons
PART I. Body Size Patterns across Space and Time
CHAPTER 1. Macroecological Patterns in Insect Body Size
Kevin J. Gaston and Steven L. Chown
CHAPTER 2. Latitudinal Variation of Body Size in Land Snail Populations and Communities
Jeffrey C. Nekola, Gary M. Barker, Robert A. D. Cameron, and Beata M. Pokryszko
CHAPTER 3. Geographic Variation in Body Size Distributions of Continental Avifauna
Brian A. Maurer
CHAPTER 4. Evolution of Body Size in Bats
Kamran Safi, Shai Meiri, and Kate E. Jones
CHAPTER 5. Macroecological Patterns of Body Size in Mammals across Time and Space
S. Kathleen Lyons and Felisa A. Smith
PART II. Mechanisms and Consequences Underlying Body Size Distributional Patterns
CHAPTER 6. Using Size Distributions to Understand the Role of Body Size In Mammalian Community Assembly
S. K. Morgan Ernest
CHAPTER 7. Processes Responsible for Patterns in Body Mass Distribution
Brian A. Maurer and Pablo A. Marquet
CHAPTER 8. The Influence of Flight on Patterns of Body Size Diversity and Heritability
Felisa A. Smith, S. Kathleen Lyons, Kate E. Jones, Brian A. Maurer, and James H. Brown
CHAPTER 9. On Body Size and Life History of Mammals
James H. Brown, Astrid Kodric-Brown, and Richard M. Sibly
Conclusion: The Way Forward
Felisa A. Smith and S. Kathleen Lyons
List of Contributors
Index