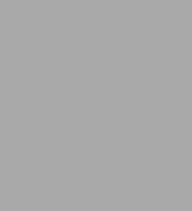
Anion Receptor Chemistry
430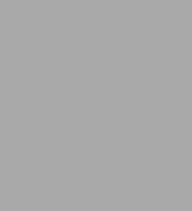
Anion Receptor Chemistry
430Hardcover
-
SHIP THIS ITEMNot Eligible for Free ShippingPICK UP IN STORECheck Availability at Nearby Stores
Available within 2 business hours
Related collections and offers
Overview
Product Details
ISBN-13: | 9780854049745 |
---|---|
Publisher: | RSC |
Publication date: | 04/07/2006 |
Series: | Monographs in Supramolecular Chemistry , #8 |
Pages: | 430 |
Product dimensions: | 6.15(w) x 9.20(h) x (d) |
Read an Excerpt
Anion Receptor Chemistry
By Jonathan L. Sessler, Philip A. Gale, Won-Seob Cho
The Royal Society of Chemistry
Copyright © 2006 The Royal Society of ChemistryAll rights reserved.
ISBN: 978-1-84755-247-1
CHAPTER 1
Introduction
1.1 Importance of Anions in the Modern World
Although often overlooked in terms of their importance, anions are ubiquitous in the natural world. Chloride anions are present in large quantities in the oceans; nitrate and sulfate are present in acid rain; and carbonates are key constituents of biomineralized materials. Anthropogenic anions, including pertechnetate, a radioactive product of nuclear fuel reprocessing, and phosphate and nitrates from agriculture and other human activities, constitute major pollution hazards.
Anions are also critical to the maintenance of life as we know it. Indeed, without exaggeration, the recognition, transport, or transformation of anions is involved at some level in almost every conceivable biochemical operation. It is essential in the formation of the majority of enzyme–substrate and enzyme–cofactor complexes as well as in the interaction between proteins and RNA or DNA. ATP, phosphocreatine, and other high-energy anionic phosphate derivatives are at the centre of power processes as diverse and important as biosynthesis, molecular transport, and muscle contraction. They also serve as the energy currency for a host of enzymatic transformations. Anion channels and carriers are involved in the transport of small anions such as chloride, phosphate, and sulfate and thus serve to regulate the flux of key metabolites into and out of cells while maintaining osmotic balance.
On a less salubrious level, mis-regulation of various anion transport mechanisms can have serious consequences. For instance, a malfunctioning of the CFTR chloride transport channel is implicated in cystic fibrosis, one of the most commonly inherited diseases among Caucasians. Likewise, the so-called ATP binding cassette transport systems, multispecific organic anion transporters, can confer resistance to a variety of modern pharmaceutical agents and are responsible in part for one of the most pressing problems in medicine, namely multidrug resistance. On very different level, an inability to process or catabolize effectively xenobiotic anions, including such chemically simple species as cyanide, oxalate, arsenate, or nitrite, can produce symptoms of chronic or acute toxicity. Poor processing of naturally occurring phosphate and sulfate is also a serious problem for patients with renal failure. Likewise, an inability to remove excess superoxide and peroxynitrite is considered responsible for many of the symptoms associated with reperfusion injury following heart attacks and strokes. On the other hand, anionic species either administered directly as in the case of fluoride used to prevent dental caries, or produced in vivo from a wide range of prodrugs, running the gamut in complexity from aspirin to AZT, are key features of modern medicine. This dichotomy underscores the complexity and importance of anion recognition in biology; it also highlights the need for, and potential utility of, synthetic anion receptor chemistry.
Later in this chapter we provide a brief selection of biologically relevant paradigms. Needless to say, in a work of this size, an exhaustive treatment is not possible. However, it is hoped that the examples chosen as highlights will help illustrate how nature uses many of the tools, such as hydrogen bonding, electrostatics, size/shape complementarily, metal–anion complex formation, and hydrophobicity, that chemists are currently employing in their efforts to achieve anion recognition. In the remainder of this chapter, we provide an historical overview that is designed both to trace the origins of the field and introduce many of the molecular recognition motifs that are continuing to play a critical role in terms of the design and synthesis of current state-of-the-art synthetic receptor systems. But first we will start with an overview of the challenges that anion complexation presents to the supramolecular chemist.
1.2 The Challenges of Anion Complexation
The design of anion receptors (and receptors for ion-pairs) is particularly challenging when compared to the design of receptors for cations. There are a number of reasons for this. Anions are larger than the equivalent isoelectronic cations (see Table 1.1) and hence have a lower charge to radius ratio. The more diffuse nature of anions means that electrostatic binding interactions are less effective than they would be for the corresponding isoelectronic cation.
Anions may be pH sensitive (becoming protonated at low pH and so losing their negative charge). Thus, receptors must function within the pH window of their target anion. This is a particular problem when designing protonated receptors for anions (e.g., ammonium containing receptors) as the protonation window of the receptor (and the anion) must also be considered. It is, of course, less of a problem for neutral receptors, or those containing permanent built-in charges, designed to operate in aprotic media.
Anionic species have a wide range of geometries (Figure 1.1) and therefore generally a higher degree of design and complementarity is required to make receptors that are selective for a particular anionic guest than for most simplecations.
The nature of the solvent in which the anion-binding event occurs plays a crucial role in controlling anion-binding strength and selectivity. Electrostatic interactions generally dominate over other recognition forces and are particularly important in stabilizing anions in solution. However, hydroxylic solvents are also noted for their ability to form strong hydrogen bonds with anions. A potential anion receptor must, therefore, compete effectively with the solvent environment in which the anion recognition event is to take place. For example, a neutral receptor that binds anions solely through hydrogen-bonding interactions is less likely to be capable of competing with the polar protic solvation shell surrounding the target anion in a hydroxylic solvent and hence may only function as an anion receptor in aprotic organic solvents (in which the anion interacts more weakly with the solvent). A charged receptor, on the other hand, can benefit from electrostatic effects and thus may compete more effectively with polar protic solvents. For example, protonated polyammonium macrocycles are capable of binding anions in water. Of course, the anion receptor must not just compete with the solvent but also with the counter cation that is necessarily paired with the targeted anion.
Ion-pairing can be very significant, particularly in non-polar solvents. Therefore, when studying anion complexation there is a necessary trade–off associated with the choice of solvent. In non-polar solvents, the anion may be weakly solvated but there may be significant ion-pairing. In more polar solvents, the solvation may be stronger – but solvation of both the cation and anion will reduce the strength of ion-pairs in solution. It is important to remember that binding experiments in solution always include an element of competition whether from solvent or from counter ion. Often these effects are ignored, but at the price of introducing what can be a significant systematic error into quantitative measurements. These errors can generally be over-looked when ostensibly similar receptor systems are being compared under analogous conditions of study. However, problems can arise when attempts are made to contrast quantitative data derived from analyses carried out using different instrumentation, or from experiments carried out in, e.g., different solvents, at different concentration regimes, or using different counter cations. Both newcomers to the field and established researcher are advised to keep these caveats in mind when trying to determine which receptor might be "best" under any particular set of conditions.
Hydrophobicity can also influence the selectivity of a receptor and, as such, any relative assessment of its anion-binding characteristics. The Hofmeister series(Scheme 1.1), which was first established through studies on the effect of salts on the solubility of proteins, orders anions by their decreasing hydrophobicity (and therefore increasing degree of aqueous solvation). Hydrophobicity may therefore be used by chemists in the design of anion receptors to bias selectivity towards larger anions with low charge. Hydrophobicity effects and the Hofmeister series are particularly relevant to the solvent extraction of anions from aqueous solution. Anion receptors that perturb (or "bias") the Hofmeister series from its normal order can allow for the selective extraction of a particular anion.
1.3 Anions in Biological Systems
Anions are ubiquitous in biology. They are present in roughly 70% of all enzymatic sites, play essential structural roles in many proteins, and are critical for the manipulation and storage of genetic information (DNA and RNA are polyanions). Anions are also involved in regulating osmotic pressure, activating signal transduction pathways, maintaining cell volume, and in the production of electrical signals. Not surprisingly, therefore, the disruption of anion flux across cell membranes (especially chloride, present in cells at the 5–15 mM concentration level) is increasingly recognized as being the primary determinant of many diseases, including cystic fibrosis, Bartter's syndrome, Dent's disease, Pendred's syndrome, and osteopetrosis. In fact, the transport of anions through cell phospholipid bilayers is known to be mediated by a variety of channels and anion transport proteins with at least 14 mitochondrial anion transport systems having been identified so far. These include (among others) systems responsible for the trafficking of ADP, ATP, phosphate, citrate, maleate, oxaloacetate, sulfate, glutamate, fumarate, and halide anions.
Recently, several X-ray crystal structures have been solved that have allowed the direct visualization of enzyme–anionic substrate complexes that are stabilized via multiple hydrogen-bonding interactions. In particular, the structure of the DNA helicase RepA sulfate complex, solved to 1.95 Å resolution, shows six hydrogen-bonding interactions between the sulfate anion and the RepA protein scaffold. The sulfate anion is also hydrogen bonded to Asp140 via an intervening water molecule (Figure 1.2).
The sulfate-binding protein (SBP) found in Salmonella typhimurium is involved in sulfate transport and consists of two globular domains that are linked by a flexible hinge. The structure of the sulfate complex of this protein, elucidated in 1985 by Pflugrath and Quiocho, reveals that the sulfate anion is bound 7 Å below the surface of the protein in a cleft between the two globular domains. When bound in this site, the sulfate anion is inaccessible to solvent. There are no charged residues present in the sulfate-binding site (Figure 1.3). Instead, the sulfate anion is bound by seven hydrogen bonds from neutral residues, five from peptide NH groups, one from a serine OH residue and one from a tryptophan NH group (Figure 1.3). The charge on the sulfate dianion is stabilized through several hydrogen bond relay systems that presumably serve to spread well into the protein matrix.
The phosphate-binding protein (PBP) is a polypeptide chain with a molecular mass of 34,400 Da. Like SBP, this protein consists of two globular domains. The phosphate-binding site is located 8 Å below the protein surface in a cleft between the two domains. The protein is involved in the transport of phosphate into bacterial cells and shows high selectivity for phosphate, binding this anion at least five orders of magnitude more strongly than sulfate. The phosphate-binding site is shown schematically in Figure 1.4. In contradistinction to SBP, the phosphate anion in PBP is bound by 12 hydrogen bonds from a combination of charged and neutral peptide residue interactions that include salt bridge involving the guanidinium group of Arg135. Anaspartate residue (Asp56 shown in blue in Figure 1.4) is thought to be responsible for the high phosphate selectivity of this protein. This residue acts as a hydrogen bond acceptor and can form a hydrogen bond with partially protonated phosphate anions. Unprotonated sulfate anions are repelled by the negative charge on Asp156. Thus, recognition depends on the protonation state of the anion and in this case is highly selective for protonated phosphates (e.g., HPO 2-4).
An X-ray crystal structure was obtained from the analysis of the histone octamer-phosphate complex, which contains fully basic phosphate anions; it revealed that five separate phosphate anions interact with lysine and arginine residues at five different sites. Figure 1.5 shows one of the phosphate ions and high-lights how it is bound by several basic amino acid residues. Both charged guanidinium (cf. the residue of arginine) and amine (the residue of lysine) are seen to interact with the phosphate anion; they do so via a combination of hydrogen bond and electrostatic interactions.
Acyclic polyamines such as spermine, NH2(CH2)3NH(CH2)4 NH(CH2)3NH2, are involved in promoting cell growth, inducing the biosynthesis of DNA, RNA, and proteins and regulating various enzymatic activities. It is also well known that polyamines display high phosphate anion affinities and bind well, for example, to phenylalanine transfer RNA. Recently, Ohishi and co-workers reported the X-ray crystal structure of a spermine–phosphate anion complex. This structure revealed that the protonated spermine interacts with the bound phosphate anion via hydrogen bonds involving intervening water molecules. In other new work, Steed and co-workers reported an X-ray crystal structure that confirmed the ability of tetraprotonated spermine binds two hydrogen phosphate anions in the solid state (Figure 1.6).
We have seen that the guanidinium group present in the active site of PBP can form salt bridges with phosphate anions. Arginine residues play a very important role in stabilising a wide range of protein polyphosphate complexes including ones involving DNA and RNA. One mode of binding that has been proposed for arginine allows it to recognize loops and bulges in RNA. RNA -binding motifs in proteins such as the human immuno-deficiency virus tat protein consist of arginine rich regions. It has been proposed that the arginine group recognizes bulges in RNA by bridging between phosphate groups that are close together in space. This binding mode, shown in Figure 1.7, has been termed "the Arginine Fork."
In contrast to the phosphate and sulfate anions, the chloride anion is spherical. It is, however, no less involved in important biological process and, not surprisingly, has been extensively studied in this context. For instance, Dijkstra and co-workers reported a 2.4 Å-resolution X-ray structure showing chloride anion bound to the active site of haloalkane dehalogenase from Xanthobacter autotrophicus GJ10. In this structure, the bound chloride is seen to interact with Trp 125 and 175 via hydrogen bond interactions with the indole NH protons (Cl···N = 3.6 and 3.2 Å, respectively). These interactions are shown in Figure 1.8. It is noteworthy that the crystals used to provide this X-ray structure were grown from a medium containing 1,2-dichloroethane. The fact that a chloride anion complex resulted thus provides important evidence that could help elucidate the catalytic mechanism that serves to convert 1-haloalkanes into the corresponding primary alcohols and a halide ion by hydrolytic cleavage of carbon–halogen bonds. On a more fundamental level this structure also provides an unequivocal proof this enzyme can interact with chloride anion. Such information is of potential practical importance since it is well known that the nitrogen-fixing hydrogen bacterium, X. autotrophicus GJ10, containing haloalkane dehalogenase degrade harmful halogenated compounds to primary alcohol with water as a co-substrate and without the need for either oxygen or ancillary co-factors.
In 2002, the 3 Å-resolution X-ray structure of the StCIC chloride ion channel was solved. This long-awaited crystal structure revealed a homodimer-derived channel that is notable for its hour glass shape and the complete absence of positively charged amino acid side chains anywhere near where the chloride anions would pass (Figure 1.9). On the other hand, the structure does indicate the presence of a negatively charged glutamate side chain just above the channel entrance. This residue is thought to act as an anion-regulating gate. By swinging out to open the channel, it allows Cl- ions to enter the channel pore from whence they are pulled (presumably) towards the constricted, neutral centre of the channel by surfaces rich in positively polarized (but not charged) residues. In spite of the elegance of this structure, and a considerable body of work devoted to understanding biological ion transport in general, our understanding of through-membrane anion transport remains extremely limited. One way of addressing this deficiency is through the synthesis and study of synthetic anion receptors and artificial anion channels, and this, in turn, is providing an important motivation to produce such systems.
The year 2002 was also marked by the exciting discovery of a new, genetically encoded amino acid, L-pyrrolysine, whose proposed biological function relies in part on pyrrole-like NH-anion recognition (cf. Figure 1.10). Pyrrole NH-chloride anion recognition and transport have also been proposed recently as an explanation for the anticancer and immunosuppressive activity displayed by prodigiosin, a naturally occurring tripyrrolic pigment known since the 1930s (vide infra). However, an alternative explanation for the observed activity has also been put forward that is based on copper complex formation and DNA cleavage. A desire to distinguish between these two limiting mechanisms and to test whether synthetic oligopyrrole species can act as anion carriers thus provides a further incentive to make and study pyrrole-based anion receptors.
(Continues...)
Excerpted from Anion Receptor Chemistry by Jonathan L. Sessler, Philip A. Gale, Won-Seob Cho. Copyright © 2006 The Royal Society of Chemistry. Excerpted by permission of The Royal Society of Chemistry.
All rights reserved. No part of this excerpt may be reproduced or reprinted without permission in writing from the publisher.
Excerpts are provided by Dial-A-Book Inc. solely for the personal use of visitors to this web site.
Table of Contents
PREFACE; CHAPTER 1; 1.1: Importance of Anions in the Modern World; 1.2: The Challenges of Anion Complexation; 1.3: Anions in Biological Systems; 1.4: Historical Overview of Synthetic Anion Receptor Chemistry; 1.5: Measurement Methods: Caveats and Limitations; 1.6: Summary Remarks; CHAPTER 2: Classic Charged Non-Metallic Systems; 2.1: Polyammoniums; 2.2: Quaternary Ammoniums; 2.3: Guanidiniums; 2.4: Amidiniums; 2.5: Imidazoliums; 2.6: Thiouronium; 2.7: Summary Remarks; CHAPTER 3: Protonated Expanded Porphyrins and Linear Analogues; 3.1: Introduction; 3.2: Cyclic Systems; 3.3: Linear Receptors; 3.4: Summary Remarks; CHAPTER 4: Neutral Non-Metallic Systems; 4.1: Amide-Based Anion Receptors; 4.2: Peptide-Based Receptors; 4.3: Urea-Based Anion Receptors; 4.4: Alcohol-Based Anion Receptors; 4.5: Hybrid Receptors; 4.6: Other Systems; 4.7: Summary Remarks; CHAPTER 5: Neutral Pyrrole Systems; 5.1: Introduction; 5.2: Cyclic Receptors; 5.3: Linear Receptors; 5.4: Summary Remarks; CHAPTER 6: Receptors for Ion Pairs; 6.1: Introduction; 6.2: Ditopic Receptors; 6.3: Cascade Complexes; 6.4: Receptors for Zwitterions; 6.5: Dual Host Extraction of Salts; 6.6: Summary Remarks; CHAPTER 7: Metal and Lewis Acid Based Receptors; 7.1: Lewis Acidic Receptors; 7.2: Metals as Organizers; 7.3: Other Anion Receptors Containing Metals; 7.4: Summary Remarks; CHAPTER 8: Sensors; 8.1: Introduction; 8.2: Devices that Employ Anion Selective Membranes; 8.3: Discrete Molecular Electrochemical Anion Sensors; 8.4: Discrete Molecular Optical Anion Sensors; 8.5: Displacement Assays; 8.6: Assays Based on Deaggregation Phenomena; 8.7: Summary Remarks; CHAPTER 9: Anion Controlled Assembly and Template-based Synthesis; 9.1: Introduction; 9.2: Halide Controlled Assemblies; 9.3: Oxyanion Directed Assemblies; 9.4: Polyfluoro-anion Directed Assemblies; 9.5: Summary Remarks; CHAPTER 10: AfterwordWhat People are Saying About This
A highly readable, concise and up-to-date overview of the exciting, vibrant and rapidly growing field of anion coordination chemistry. As world leading practitioners in the area, the authors' enthusiasm for the subject matter comes across loud and clear.