Apoptosis: The Life and Death of Cells available in Paperback
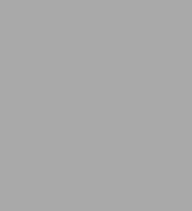
Apoptosis: The Life and Death of Cells
- ISBN-10:
- 052162679X
- ISBN-13:
- 9780521626798
- Pub. Date:
- 08/16/2004
- Publisher:
- Cambridge University Press
- ISBN-10:
- 052162679X
- ISBN-13:
- 9780521626798
- Pub. Date:
- 08/16/2004
- Publisher:
- Cambridge University Press
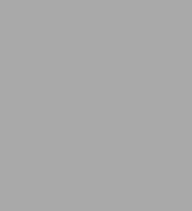
Apoptosis: The Life and Death of Cells
Paperback
Buy New
$54.99Buy Used
$54.18-
-
SHIP THIS ITEM
Temporarily Out of Stock Online
Please check back later for updated availability.
-
Overview
Product Details
ISBN-13: | 9780521626798 |
---|---|
Publisher: | Cambridge University Press |
Publication date: | 08/16/2004 |
Series: | Developmental and Cell Biology Ser. |
Edition description: | New Edition |
Pages: | 228 |
Product dimensions: | 7.01(w) x 10.00(h) x 0.47(d) |
Read an Excerpt
Cambridge University Press
0521622719 - Apoptosis - The Life and Death of Cells - by Christopher Potten and James Wilson
Excerpt
Dead or alive?
How do we know whether a cell is dead or alive? This question is somewhat analogous to the difficult ethical and philosophical questions that doctors face in defining the point of death of a human. In order to consider this question we need first to determine what we understand by the term living. Conventionally, life is generally defined by an organism being able to demonstrate various activities. Broadly speaking, these can be divided into four categories as follows:
(a) movement,
(b) metabolism,
(c) sensory perception, and
(d) reproduction.
These properties shall now be considered individually.
Movement
Most living organisms exhibit movement relative to their environment, to a greater or lesser degree. There are some apparently sedentary organisms, but even in these some levels of movement are generally detectable. For example, many plants, although fixed at a particular position in space display phototropism and can track the movement of the sun throughout the day. Plants are also geotropic and their roots grow (move) downwards in response to gravity, whereas their shoots show phototropism and grow upwards in response to light. Within the mammalian body, considerable evidence of individual cellular movement can be found. This ranges from the movement of cells through tissues as they mature and differentiate into functional cells to the passive movement of circulating red blood cells to active migration of leukocytes into and within tissues in response to chemotactic signals to, of course, the highly active and directional swimming of sperm in response to chemical signals.
In addition to this movement of organisms in three-dimensional space and the movement of cells within the three-dimensional structures of tissues, there is clear evidence of intracellular movement, that is, the movement of organelles within the body of the cell (seen clearly in plants, fungi, and animals). These movements, such as the 'flow' of mitochondria through the cytoplasm, can be readily observed using time-lapse video microscopy. This type of movement within a cell is somewhat analogous to the movement of blood through the blood vessels in a multicellular, multitissued animal. Movement, whether of individual cells or whole organisms, clearly involves extensive coordination of inter- and intracellular mechanisms and cellular skeletal elements that permit movement. These require biochemical reactions and ultimately the transcription and translation of genes.
Metabolism
All living organisms, including individual cells in the tissues of the body, have to undertake biochemical, metabolic processes in order to convert nutrient materials into useful cellular products and to process waste by-products for excretion. In humans, this metabolic activity requires oxygen uptake and utilisation, with carbon dioxide being produced as a waste product, whereas plants take up and utilise carbon dioxide and excrete oxygen. This is one clear reason why we are so completely dependent on our botanical co-inhabitors of this planet. Cellular metabolism provides essential materials (molecules) required for the correct functioning of the cell, tissue, or organism as a whole and for an individual cell to grow and increase its mass or for cells to divide and multiply to increase the mass of a tissue, that is, for tissue or body growth. It is relatively easy to see how these metabolic activities can be considered part of a definition of life for individual cells, as well as multicellular organisms like humans.
Sensory perception
Another fundamental property of life is the ability to perceive and respond to environmental signals. Plants respond to light, gravity, temperature, nutrient and water supplies. Animal cells and organisms respond to much the same types of stimuli. Therefore, both cells and organisms must have mechanisms for detecting the environment (i.e., sensory cells or tissues) and an ability to respond to the changes that they detect. Once again, these processes require the coordination of complex intra- and extracellular programmes.
Reproduction
The ability of cells to reproduce themselves is fundamental to the life of multicellular organisms and the ability of whole organisms to reproduce (divide) is essential to the survival of all life-forms. Bacteria may reproduce by simply dividing in two. In more complex organisms such as mammals, cells reproduce in a similar way, by a process called mitosis. Increasing cell numbers through division contributes to the growth of tissues, along with increased mass and volume of individual cells.
Most living organisms also reproduce sexually, by producing a special form of reproductive or germ cell by a special type of cell division, termed meiosis, which results in the production of male and female germ cells, sperm and ova, each of which has half the normal, full cellular DNA content (i.e., for humans a single copy of each of the twenty-three chromosomes). When germ cells are brought together, they fuse to form a new, single-celled organism (the fertilised zygote) from which the highly complex multicellular organism will develop via repeated acts of cell division. Before mitotic cell division can occur the genetic material of the cell, the DNA, must be replicated so that immediately prior to mitosis, the cell contains a double complement of DNA (four copies of each chromosome for humans).
In addition to sexual reproduction, there are other forms of vegetative or nonsexual procreation. These may involve the process of budding off a new individual or other acts that amount to cloning, where offspring are generated that are identical to the parent (i.e., the growth of new plants or animals from severed segments (cuttings) of the parent. Such asexual reproduction fails to generate genetic diversity and as a consequence is not beneficial in adverse or altered environmental conditions where natural selection is advantageous.
As stated previously, for a cell to reproduce itself (i.e., divide) it must first reproduce its genetic material, the DNA, and other essential, subcellular elements. Each human cell has about two metres of DNA coiled within its nucleus. This amount of DNA can be accommodated within a cell's nucleus by virtue of its specialised structure. DNA has a double-helix structure and is complexed and coiled up with other molecules (these include proteins called histones) to form chromosomes. In humans there are twenty-three different chromosomes, with most cells having one pair of each (i.e., forty-six chromosomes). The genetic code relies on the appropriate arrangement of the DNA bases, adenine, guanidine, cytosine, and thymidine (A, G, C, and T, respectively), which form the coded messages of the individual genes that control all cellular activity. To copy the genetic code of the DNA the chromosomes must be uncoiled (an extremely complex and not fully understood process). The synthesis, or replication, of DNA is very complex and must be completed without errors, which would otherwise be multiplied in the successive generations of cells: genetic errors are the basis of diseases such as cancer. The DNA must then be recoiled and condensed back into the chromosome structures that may then be separated by the process of cell division or mitosis. These sequential processes of DNA uncoiling, replication, recondensation, and cell division are collectively termed the cell cycle and are described in greater detail in Chapter 4 of this book.
The ability of a cell to reproduce itself via division (mitosis) is commonly used as a measure of cell viability in many studies where toxic drugs, chemicals, or radiation are, for example, assessed for their ability to kill cancer cells. This cellular reproduction is alternatively referred to as cellular proliferation. A cell may fail to divide successfully because the DNA, or other critical elements of the cell, have been damaged in such a way as to prevent DNA replication or cell division. The damaged cell may then undergo degradative changes. If the damage is particularly severe the cell will swell and lyse, spilling out its contents, a process of cell death called necrosis. Alternatively, the cell may activate a programme of genetic and biochemical changes to control its own death - suicide, if you like; this process is termed apoptosis. This involves shrinkage of the cell and its fragmentation. The fragments of the cell may then be engulfed and digested by other cells. Genetically programmed and temporally controlled cell death, termed programmed cell death, was well recognised in developing embryos prior to the description of apoptosis in the scientific literature. Apoptosis and programmed cell death are essentially variations on a theme and the terms are used interchangeably throughout many scientific publications.
It may be that a cell will attempt to undergo mitosis but because of damage to the DNA (or other cellular structures) it is incapable of exiting this phase of the cell cycle and by default dies by apoptosis. This is a process we shall call here mitotic cell death (see 'Mitotic death' in Chapter 2).
Finally, cells may also fail to divide when they switch from proliferation to differentiation, that is, they mature and take on a specific functional role. The fact that a fully differentiated, functional cell does not replicate its DNA and does not divide means that a certain level of DNA damage may be tolerated, as the stringent checks for DNA errors that occur during the cell cycle are not applied or needed. Differentiation can be regarded as the process, or series of steps, that leads to the production of a functional or differentiated cell (defined in greater detail in Chapter 4). Typical differentiated cells are red blood cells, the cells on the outer surface of your skin, muscle and nerve cells, and cells that secrete products such as mucus, enzymes, saliva, and milk.
Full or terminal differentiation is usually incompatible with further rounds of cell division. Cells that do not obviously fall into this category may, nevertheless, express a spectrum of limitations in terms of their division potential. These may range from their ability to divide once after exposure to a cytotoxic agent within a certain time frame to being able to divide effectively indefinitely. To study this aspect of cell survival, compromises have to be made so that one can define a series of criteria that can be used in an objective fashion. Such compromises are common in scientific experimentation.
The convention that has been adopted here is that cells are said to survive after a particular treatment (i.e., be alive), if they are capable of undergoing about six cell divisions or are capable of producing a minimum of fifty daughter cells within a prescribed time limit. An obvious compromise here is that six divisions should in theory give sixty-four cells, if each one divided on every occasion. For convenience, this is commonly reduced to the figure of fifty daughter cells produced. These studies are easy to perform using cell culture systems, where each daughter cell may remain attached to each other and form a colony, which because they are derived from a single surviving cell are also referred to as clones. The single cells that give rise to the colony are called colony forming cells or clonogenic cells. Clones here have a slightly different connotation from the current debate on cloning animals such as sheep, mice, and humans, where the animal is being derived from a single manipulated cell (as discussed), but here it is required to generate a diversity of differentiated tissues and many billions of cells.
These experimental approaches are essentially designed to study cells that survive a particular treatment by the maintenance of their reproductive potential and the cells that die, by definition, are those that lose their reproductive potential or become sterilised. One issue here is that, within limits of a set time frame, some cells may not satisfy the criteria because they are reproducing too slowly but if a longer time frame was used they also would be capable of producing fifty cells. Another complexity is that the cells may not always divide in a truly exponential fashion, that is, one cell producing two, two producing four, and so on, but the tissue or colony may be organised in a more complex fashion with cells entering differentiation and different categories of proliferating or reproducing cells (cell lineages or cell hierarchies; see Chapters 6 & 7).
Cell death: human analogies
So, if metabolism, movement, sensory perception, and reproduction are the basic features of life, death must be characterised by the absence of these features. Is it necessary for all these processes to cease for death to be defined? Cells may stop movement but still be capable of metabolism; they may stop reproduction and still be capable of metabolism and movement; they may stop reproduction and movement but still be metabolically active. The difficulty one encounters here is very similar to the modern ethical and philosophical problems associated with defining the point of death for a human. It might be worthwhile to digress for a moment and consider these questions in somewhat greater detail.
If an individual capable of movement suddenly ceases to move, a concern might arise as to whether death has occurred; however, the individual may simply be asleep (from a cellular point of view, in a dormant state). Careful examination would reveal a persistence of autonomic, involuntary movement, for example, the chest moving as a consequence of breathing, evidence of blood circulation, and eye movements. The presence of such movements somewhat alleviates the immediate anxiety as to whether death has occurred. However, the individual could be in a coma; now the situation is more serious. Breathing still occurs, movement of the blood around the vascular system continues, and the tests for whether life exists become somewhat more stringent. Does the individual respond to sensory perception? For example, does a person wake up if he or she is pricked with a needle? Is the main sensory organ, the brain, functioning properly? In fact, different levels of brain function may need to be determined: cerebral activity, brain stem function, and so on. Such situations also inevitably lead one to address the following question: 'Can metabolic activity still be demonstrated?' Obvious food intake may have ceased for the individual but questions arise as to whether the person is still taking up oxygen (breathing), still excreting waste (full kidney function), and so on.
Failure of any one of the major organs of the body can precipitate death. The kidneys may fail and the levels of blood waste products, most noticeably urea, will rise. The liver may fail and because it is a major metabolic organ many other toxins can be built up in the blood. The lungs may fail and the blood gas levels will change. All of these are easily detected by appropriate blood tests but if not dealt with can prove fatal. One can suffer an aneurysm of the main aorta as a consequence of cholesterol buildup; effectively the aorta bursts. There can be various defects associated with the heart, ranging from ventricular fibrillation (where failure to pump blood properly results in the brain not receiving enough oxygen) to coronary thrombosis or myocardial infarction, which are essentially characterised by a blocking of the blood supply to various regions of the heart, producing the symptoms known as a heart attack. Some of these defects can be detected by checking the pulse, using a stethoscope or, ultimately, using an electrocardiogram (ECG), which analyses the contraction of the heart. Finally, there are various problems associated with the brain and the brain stem that can result in rupture of blood vessels from head injury, or from infarction caused by clots in the blood vessels supplying the brain. Both these events result in starving the brain first of blood, ischaemia, and, consequently, oxygen (hypoxia). The severity of brain injury depends on the size and the location of the ischaemic area and the consequences are the symptoms known as a stroke. There are a whole variety of levels of unconsciousness or coma that can occur that are generally tested for by looking for electrical activity in the brain using an electroencephalogram (EEG). The most severe state would give little or no reading on an EEG for the brain cortex, but if there is still brain stem function then breathing and heart activity and one or two basic reflexes will continue to be detected. However, such a person would be in a persistent vegetative state and would require life support systems.
Now, a feature of all these defects in the human body is that, provided that the patient can receive adequate medical treatment and can fairly rapidly be taken to hospital, many of them can be overcome in modern medical practice by the use of appropriate equipment: kidney dialysis machines, heart-and-lung machines, and so on. So although these individuals would have undoubtedly died if they had not received appropriate treatment, they can be rescued from death by modern techniques. People on dialysis machines, those fitted with heart pacemakers, and so on, can lead a relatively normal life. The most difficult cases are those in coma, particularly those in a persistent vegetative state, and the question to what extent such individuals can be regarded as alive remains a matter of some argument. Once brain stem activity is lost, although a patient can be kept technically "undead" on life support systems, switching off these systems will inevitably lead directly to death. Major legal and ethical questions arise in these cases. The difficulties outlined here can also be considered in relation to single cells in tissues or in a culture dish.
In modern medicine the ethical problems arise because a person can lose many of the key functions of life and yet be kept "alive" by appropriate machines. The kidneys may have failed, the lungs may have ceased functioning, and the heart may even have stopped pumping oxygen around the body, but the brain may still be capable of some function and the individual cells of the body are clearly still alive. The individual cells are still metabolically and reproductively active and will function normally if transplanted into another individual. So, at what point does one define death in this sort of situation?
One or two real-life examples may reinforce the difficulties to which I allude. At the 1989 Hillsborough football stadium disaster in the United Kingdom, many people were crushed and a few were unconscious when taken into hospital, where they were kept in a persistent vegetative state by intensive maintenance theory. Eventually the courts ruled that in one case support systems could be switched off, that is, the final stages of dying were permitted to occur. However, another patient from the same accident in a similar vegetative state continued to receive supportive treatment and eventually was able to communicate again, at least to a limited extent.
An often-quoted case concerns a man from Minnesota involved in a serious car accident in California in 1978. He required several blood transfusions after the accident. He remained unconscious and could not maintain unaided respiration. No next of kin could be traced and a transplant team was notified because his heart and kidneys were undamaged. Appropriate consultations took place and in California he was legally pronounced brain dead. However, some time later a son arrived and legal action was taken, based on the fact that in Minnesota brain death was not recognised. So in one state in the United States he was legally defined as dead, whereas in another he was legally defined as alive at the time the ventilator was switched off and his organs were removed. Such cases illustrate the difficulties, both legal and ethical, in defining death.
Similar complications arise when we define death as loss of reproductive potential. Clearly for adult humans, the question of whether they can still reproduce is not generally considered part of the definition of life or death. Women beyond the age of menopause are not categorised as dead! But for cells this is a fairly common criterion by which their full functional competence is assessed, and the loss of reproductive ability is regarded as a serious marker of loss of cellular functional activity and hence cell death.
These considerations apply also to individual cells, whether they are free living or part of the tissues of the body, and one is forced to consider the following question: 'At what point does a cell die and loss of which functions are most appropriate to use to define the loss of life?' Cells can clearly enter phases of dormancy or inactivation during which most of the functions of life might be regarded as being absent (extreme examples are seeds or spores). These cells can be brought back to life after even long periods and harsh environmental conditions by providing the right signals or environment. Cells can become quiescent in tissues of the body when they do not continue to progress through the sequential metabolic processes leading to cell division. They may slow down or stop their movement, relative not only to other cells in the tissue but also to the internal movement of the cytoplasm. They might become much more resistant to various changes in the environment (loss of sensory responses) and certainly they may cease to reproduce.
It is therefore important to remember that death is not as simple a state to define as we might at first imagine. Of course, once true death has occurred it may be very easy to recognise and we discuss this further when we consider the appearance of dying or dead cells. Again, it is necessary to think of the human situation - only when it becomes very obvious that once death has really occurred is it easy to recognise. The body starts to dehydrate, decay, and disintegrate.
© Cambridge University Press