Climate Change: Causes, Effects, and Solutions / Edition 1 available in Paperback
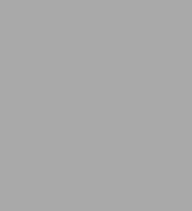
Climate Change: Causes, Effects, and Solutions / Edition 1
- ISBN-10:
- 0470850191
- ISBN-13:
- 9780470850190
- Pub. Date:
- 06/27/2003
- Publisher:
- Wiley
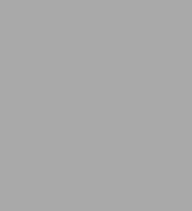
Climate Change: Causes, Effects, and Solutions / Edition 1
Buy New
$87.95Buy Used
$61.90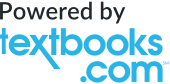
-
SHIP THIS ITEM— This item is available online through Marketplace sellers.
-
PICK UP IN STORECheck Availability at Nearby Stores
Available within 2 business hours
This item is available online through Marketplace sellers.
-
SHIP THIS ITEM
Temporarily Out of Stock Online
Please check back later for updated availability.
This item is available online through Marketplace sellers.
Overview
Climate Change: Causes, Effects and Solutions examines the latest scientific findings without any advanced technical knowledge. It goes beyond a description of changes in the physical environment to consider the broader issues of ecological, economic and human effects of climate change.
The book explains:
- the causes and effects of climate change from a natural and human environment perspective.
- mitigation options and policies that could reduce the impacts of climate change.
- global impacts - with case studies are taken from North America, Europe, Australasia and elsewhere.
Essential reading for undergraduates and general readers who want to heighten their knowledge and understanding of this important problem.
Product Details
ISBN-13: | 9780470850190 |
---|---|
Publisher: | Wiley |
Publication date: | 06/27/2003 |
Pages: | 272 |
Product dimensions: | 7.44(w) x 9.69(h) x 0.61(d) |
About the Author
Read an Excerpt
Climate Change
Causes, Effects, and SolutionsBy John T. Hardy
John Wiley & Sons
Copyright © 2003 John Wiley & Sons, LtdAll right reserved.
ISBN: 0-470-85018-3
Chapter One
Earth and the Greenhouse Effect
"... if the carbonic acid content of the air [atmospheric C[O.sub.2]] rises to 2 [i.e. doubles] the average value of the temperature change will be ... +5.7 degrees C" Svante Arrhenius 1896
Introduction
The physics and chemistry of the Earth's atmosphere largely determines our climate (Lockwood 1979). Although the atmosphere seems like a huge reservoir capable of absorbing almost limitless quantities of our industrial emissions, it is really only a thin film. Indeed, if the Earth were shrunk to the size of a grapefruit, its atmosphere would be thinner than the skin of the grapefruit. Our understanding of how the chemistry and physics of the atmosphere affect climate developed over many centuries, but has greatly accelerated during the past few decades (Box 1.1).
The Earth's atmosphere is layered. In the lower atmosphere, from the surface up to about 11-km altitude (troposphere), temperature decreases with increasing altitude. This layer is only about 1/1,200 of the diameter of the globe, but its physics and chemistry are crucial to sustaining life on the planet. Because cold dense air on top of warm less dense air is unstable, the layeris fairly turbulent and well mixed. It contains 99% of the atmospheric mass. From 15 to 50 km, the temperature increases with altitude, resulting in a stable upper atmosphere (stratosphere) with almost 1% of the atmospheric mass. Above 50 km are the mesosphere and the thermosphere, which have little effect on climate (Figure 1.1).
During the past 100 years we humans, as a result of burning coal, oil, and gas and clearing forests, have greatly changed the chemical composition of this thin atmospheric layer. These changes in chemistry, as described in subsequent chapters, have far-reaching consequences for the climate of the Earth, the ecosystems that are sustained by our climate, and our own human health and economy.
The Greenhouse Effect
Three primary gases make up 99.9% by volume of the Earth's atmosphere - nitrogen (78.09%), oxygen (20.95%), and argon (0.93%). However, it is the rare trace gases, that is, carbon dioxide (C[O.sub.2]), methane (C[H.sub.4]), carbon monoxide (CO), nitrogen oxides (N[O.sub.x]), chlorofluorocarbons (CFCs), and ozone ([O.sub.3]) that have the greatest effect on our climate. Water vapor, with highly variable abundance (0.5-4%), also has a strong influence on climate. These trace gases are known as greenhouse gases or radiatively important trace species (RITS). They are radiatively important because they influence the radiation balance or net heat balance of the Earth.
Thermonuclear reactions taking place on our nearest star, the Sun, produce huge quantities of radiation that travel through space at the speed of light. This solar radiation includes energy distributed across a wide band of the electromagnetic spectrum from short-wavelength X rays to medium-wavelength visible light, to longer-wavelength infrared. The greatest amount of energy (44%) is in the spectral region, visible to the human eye from 0.4 (violet) to 0.7µm (red) (Figure 1.2a).
As incoming solar radiation passes through the atmosphere, particles and gases absorb energy. Owing to its physical or chemical structure, each particle or gas has specific wavelength regions that transmit energy and other regions that absorb energy. For example, ozone in the stratosphere absorbs short- and middle-wavelength ultraviolet radiation. A large percentage of incoming solar radiation is in the visible region. Atmospheric water vapor, carbon dioxide, and methane have low absorption in this region and allow most of the visible light to reach the Earth's surface.
After absorption by the Earth's surface, visible energy is transformed and radiated back in the far-infrared (heat) region of the spectrum at wavelengths greater than 1.5µm (Figure 1.2b). The transparency of the atmosphere to outgoing far-infrared radiation (heat) determines how much heat can escape from the Earth back into space and how much is trapped. The important feature of greenhouse gases is that they absorb (are opaque to) certain infrared wavelengths. Water vapor, carbon dioxide, and methane, the same gases that transmit visible wavelengths, absorb strongly in the far infrared (Figure 1.2b). Thus, they trap heat in the troposphere and stop it from escaping to space. Window glass used to trap heat in a greenhouse has similar absorption and transmission properties; hence, the term "greenhouse gases."
Of the total amount of solar energy reaching the Earth's atmosphere (342 W [m.sup.-2]), an average 31% is reflected back to space by the upper surface of clouds, the particles in the atmosphere (dust and aerosols), or the surface of the Earth (Figure 1.3). While the overall average reflectivity (also called albedo) of the Earth is 31%, albedo differs greatly between surfaces. Clouds, with an albedo of 40 to 90%, are by far the most important reflectors of incoming solar radiation. The albedo of fresh snow is 75 to 90%, forests 5 to 15%, and that of water, which depends on the angle of inclination of the Sun, ranges from 2 to >99%. Incoming energy that is not reflected (the remaining 69%) is absorbed by the troposphere and the Earth's surface (Figure 1.3). Evaporation of water requires a considerable amount of energy. This energy is essentially stored as "latent heat" in water vapor and released back into the air as heat when water vapor condenses (Figure 1.3).
Of the total far-infrared (heat) energy reradiated from the Earth's surface, 83% is back-radiated and does not directly escape the atmosphere. This back radiation is about double the amount of energy absorbed by the surface directly from the Sun. This additional atmospheric energy warms the Earth to its present temperature. Without the greenhouse warming effect of the atmosphere, the Earth's average surface temperature would be about -20ºC (-4ºF) instead of 15ºC (59ºF). Simply put, greenhouse gases trap solar heat in the lower atmosphere and keep the Earth warm. As long as the amount of incoming solar energy and the amount of greenhouse gas in the atmosphere remain fairly constant, the Earth's temperature remains in balance (Figure 1.3). However, the greater the concentration of greenhouse gases, the greater the amount of long-wave (heat) radiation trapped in the lower atmosphere.
Large-Scale Heat Redistribution
The Earth's temperature is not uniform but differs greatly - geographically (horizontally), by elevation (vertically), and over time (seasons and decades). To understand climate and how it might change over decades to centuries in the future, we need to understand how heat is distributed over the Earth. The tropics are warmer than temperate or polar regions because incoming solar radiation strikes the Earth's surface at a greater angle of incidence, resulting in more radiation per unit surface area (Figure 1.4). The same energy striking higher-latitude areas is distributed over a greater surface area. Thus, the radiation balance of the Earth is unevenly distributed and the excess heat from the tropics will (according to the laws of thermodynamics) tend to redistribute itself poleward to cooler areas. This occurs as direct heat transfer by the poleward movement of warm air and water masses. Also, significant heat energy, used in evaporation of surface water in the tropics, is carried as latent heat and released at higher latitudes when water vapor cools and condenses into rainfall.
The initial uneven distribution of heat and density in the atmosphere and ocean results in important large-scale circulation patterns (Figure 1.5). Warm air near the equator rises, and cools as it flows poleward at high altitude. At about 30º latitude, it sinks and flows southward again at lower altitudes. To replace the sinking air mass, air is drawn from polar latitudes, flows south at high altitudes, sinks, and then flows poleward near the surface. These patterns are modified by the Coriolis force, which pushes the circulation clockwise in the Northern Hemisphere and counterclockwise in the Southern Hemisphere (Box 1.2). This results in major surface wind patterns at lower altitudes including, for example, in the Northern Hemisphere, the temperate westerly and northeast trade winds (Figure 1.5).
In the ocean, wind patterns, along with density (salinity) differences drive the major circulation currents such as the Gulf Stream in the North Atlantic and the Koroshio Current in the North Pacific. Huge quantities of heat are transported with the surface currents from south to north in the Western Atlantic and Pacific Oceans by what has been termed the oceanic conveyor belt (Figure 1.6). Thus, major ocean and atmosphere circulation patterns are closely tied to the Earth's heat balance and any disruption of these patterns could cause rapid changes in global climate.
Greenhouse Gases
Historically, greenhouse gas concentrations in the Earth's atmosphere have undergone natural changes over time and those changes have been closely followed by changes in climate. Warmer periods were associated with higher atmospheric greenhouse gas concentrations and cooler periods with lower greenhouse gas concentrations. However, those changes were part of natural cycles and occurred over periods of tens of thousands to millions of years (Chapter 2). Recent human-induced changes in atmospheric chemistry have occurred over decades (Ramanathan 1988). When referring to the postindustrial era, scientists generally use the term climate change in the way defined by The UN Framework Convention on Climate Change. Thus, "climate change" is a change of climate that is attributed directly or indirectly to human activity that alters the composition of the global atmosphere and which is, in addition to natural climate variability, observed over comparable time periods.
Human activities generate several different greenhouse gases that contribute to climatic change. To determine the individual and cumulative effects of these gases on the Earth's climate, we need to examine their total quantity, their natural and human sources to the atmosphere, their rates of loss to natural sinks, their past and projected rates of increase, and their individual and cumulative heating capacities (Table 1.1).
Water vapor traps heat in the atmosphere and makes the greatest contribution to the greenhouse effect. Its level in the atmosphere is not directly the result of human activities. However, because warmer air can hold more water vapor, an increase in the Earth's temperature resulting from other greenhouse gases produces a "positive feedback," that is, more warming means more water vapor in the atmosphere, which in turn contributes to further warming (Chapter 3).
Carbon dioxide is a natural component of the atmosphere and is very biologically reactive. It can be reduced to organic carbon biomass through photosynthetic uptake in plants and, through biological oxidation (respiration), converted back to gaseous C[O.sub.2] and returned to the atmosphere. Major natural sources to the atmosphere are animal respiration, microbial breakdown of dead organic matter and soil carbon, and ocean to atmosphere exchange (flux). Sinks include photosynthetic uptake by plants and atmosphere to ocean flux. These natural cycles maintained the atmospheric concentration of C[O.sub.2] at about 280 ± 10 ppmv (parts per million by volume) for several thousand years prior to industrialization in the mid-nineteenth century.
During the past 150 years, and especially during the last few decades, humans greatly increased the concentration of atmospheric C[O.sub.2]. Huge reservoirs of carbon, stored for millions of years as fossilized organic carbon (coal, oil, and gas) in the Earth's crust, have been removed and burned for fuel. When carbon fuels burn, they combine with atmospheric oxygen to produce carbon dioxide, which enters the atmosphere (Figure 1.7). Globally, more than 80% of human C[O.sub.2] emissions come from transportation and industrial sources. The remaining 20% comes primarily from deforestation and biomass burning. A forest stores about 100 tons of carbon per acre and about half the world's forest was destroyed in the last half of the twentieth century. Carbonate minerals used in cement production also release C[O.sub.2] to the atmosphere. These sources altogether contribute 6.5 billion tons or gigatons of carbon (GtC) to the atmosphere each year (Figure 1.7). The largest emitters of C[O.sub.2] are the United States, China, and the Russian Federation (Figure 1.8). Furthermore, the rate of addition to the atmosphere from these sources exceeds the rate of loss to major C[O.sub.2] sinks by about 3.3 GtC per year (Box 1.3, Figure 1.9). Thus, the atmospheric concentration of C[O.sub.2] continues to increase.
The GWP of atmospheric carbon dioxide is not a new concept, but dates back to the nineteenth century. In 1896, the Swedish chemist Svante Arrhenius estimated that fossil-fuel burning would result in a doubling of the atmospheric C[O.sub.2] concentration 3,000 years in the future (Arrhenius 1896). He correctly described the process, but severely underestimated the time frame in which humans could double the atmospheric C[O.sub.2] concentration. By 1938 some scientists concluded that human combustion of fossil fuel was already leading to a significant increase in atmospheric C[O.sub.2] and global average temperature (Callendar 1938).
By the end of the twentieth century, the atmospheric concentration of C[O.sub.2] had risen to over 367 ppmv - an increase of 31% above its preindustrial level - and it continues to increase exponentially at about 0.5% per year (Figure 1.10). The present atmospheric C[O.sub.2] concentration has not been exceeded during the past 420,000 or perhaps even 20 million years (Houghton et al. 2001). Even if current C[O.sub.2] emissions are reduced and maintained at or near 1994 rates, the atmospheric concentration will reach 500 ppmv (nearly double the preindustrial level) by the end of this century - far sooner than Arrhenius could have imagined. The major long-term reservoir (sink) for C[O.sub.2] is the deep ocean. Carbon dioxide produced today will take more than 100 years to be absorbed by this reservoir. Thus, even if all emissions ceased today, atmospheric C[O.sub.2] would remain above its preindustrial level for the next 100 to 300 years.
Fossil fuels are nonrenewable and their supply is finite. However, current supplies are abundant, relatively inexpensive, and could last for another 40 to 200 years (Table 1.2).
Continues...
Excerpted from Climate Change by John T. Hardy Copyright © 2003 by John Wiley & Sons, Ltd . Excerpted by permission.
All rights reserved. No part of this excerpt may be reproduced or reprinted without permission in writing from the publisher.
Excerpts are provided by Dial-A-Book Inc. solely for the personal use of visitors to this web site.
Table of Contents
Preface.Section I Climate Change – Past, Present, and Future.
1. Earth and the Greenhouse Effect.
Introduction.
The Greenhouse Effect.
Large-Scale Heat Redistribution.
Greenhouse Gases.
Warming Potentials.
Summary.
2. Past Climate Change: Lessons From History.
Introduction.
Past Climate Change – Six Historic Periods.
Methods of Determining Past Climates and Ecosystems.
Rapid Climate Change.
Lessons of Past Climate Change.
Summary.
3. Recent Climate Change: The Earth Responds.
Introduction.
Atmospheric Temperatures.
Water Vapor and Precipitation.
Clouds and Temperature Ranges.
Ocean Circulation Patterns.
Snow and Ice.
Sea-Level Rise.
Animal Populations.
Vegetation.
Attribution.
Summary.
4. Future Climate Change: The Twenty-First Century and Beyond.
Introduction.
Global Climate Models.
Feedback Loops and Uncertainties.
Scenario-Based Climate Predictions.
Regional Climates and Extreme Events.
The Persistence of a Warmer Earth.
Summary.
Section II Ecological Effects on Climate Change.
5. Effects on Freshwater Systems.
Introduction.
Surface and Groundwater.
Drought and Soil Moisture.
Lake and Stream Biota.
Human Infrastructure.
Wetlands.
The Cryosphere.
Managing Water.
Summary.
6. Effects on Terrestrial Ecosystems.
Introduction.
Geographic Shifts in Terrestrial Habitats.
Vegetation-Climate Interactions.
Effects of Disturbances.
Loss of Biodiversity.
Implications for Forest Management and Conservation Policy.
Summary.
7. Climate Change and Agriculture.
Introduction.
Effects of Agriculture on Climate Change.
Effects of Climate Change on Agriculture.
US Agriculture.
Global Agriculture.
Summary.
8. Climate Change and the Marine Environment.
Introduction.
Sea-Level Rise.
Ocean Currents and Circulation.
Marine Biogeochemistry.
Marine Ecosystems.
Summary.
Section III Human Dimensions of Climate Change.
9. Impacts on Human Settlement and Infrastructure.
Introduction.
Energy.
Environmental Quality.
Extreme Climatic Events.
Human Settlements.
Infrastructure.
Summary.
10. Effects of Climate Change on Human Health.
Introduction.
Direct Effects of Heat Stress.
Infectious Diseases.
Air Quality.
Interactions and Secondary Effects.
Summary.
11. Mitigation: Reducing the Impacts.
Introduction.
Capture or Sequester Carbon Emissions.
Reduce Global Warming or Its Effects by Geoengineering.
Enhance Natural Carbon Sinks.
Convert to Carbon-Free and Renewable Energy Technologies.
Conserve Energy and Use It More Efficiently.
Adapt to Climate Change.
Taking Action.
Summary.
12. Policy, Politics, and Economics of Climate Change.
Introduction.
International Cooperation – From Montreal to Kyoto.
Meeting Kyoto Targets.
Post-Kyoto Developments.
The Politics of Climate Change.
Kyoto Without the United States.
Benefits and Costs of Mitigating Climate Change.
The Future – What is Needed?
Summary.
Appendix A: Units.
Appendix B: Abbreviations and Chemical Symbols.
Appendix C: Websites on Climate Change
Index.