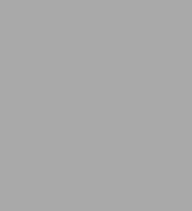
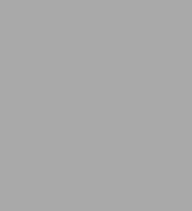
Hardcover
-
SHIP THIS ITEMNot Eligible for Free ShippingPICK UP IN STORECheck Availability at Nearby Stores
Available within 2 business hours
Related collections and offers
Overview
Read an Excerpt
Cyclodextrins in Chromatography
By Tibor Cserháti, Esther Forgács
The Royal Society of Chemistry
Copyright © 2003 The Royal Society of ChemistryAll rights reserved.
ISBN: 978-0-85404-540-2
CHAPTER 1
Chemistry and Physicochemistry of Cyclodextrins
The main products of photosynthesis are two glucose polymers: cellulose and starch. These two carbohydrate polymers represent a considerable part of the renewable energy source of the Earth. While cellulose is the resistant and structure-forming component in the cells, starch is the mobilisable, convertible energy depot. Starch consists of two glucose polymers: amylose and amylopectin. Dextrins, the products of partial hydrolysis of starch, can give cyclic dextrins under the action of glucosyltransferase enzyme. Cyclodextrins (CDs) are cyclic, non-reducing oligosaccharides consisting of D-glucopyranose units bonded through α-1,4 linkages.
The three major cyclodextrins are the following; the smallest is the α-CD (Schardinger's α-dextrin, cyclomaltohexose, cyclohexaglucan, cyclohexaamylose or C6A) with six glucose units, followed by β-CD (Schardinger's β-dextrin, cyclomaltoheptose, cycloheptaglucan, cycloheptaamylose or C7A) with seven glucose units and γ-CD (Schardinger's γ-dextrin, cyclomaltooctose, cyclooctoglucan, cyclooctaamylose or C8A) with eight glucose units. Unsubstituted native CDs are crystalline, non-hygroscopic, homogeneous substances, which are torus-like macrocycles built up from glucopyranose (glucose) units (Figure 1.1). In CDs, the sugars adopt a 4C1 chair conformation and orient themselves so that the molecule forms a toroidal truncated cone structure. The cavity is lined by the hydrogen atoms and the glycosidic oxygen bridges. The non-bonding electron pairs of the glycosidic oxygen bridges are directed towards the inside of the cavity to produce a high electron density and lend it some Lewis base character.
The C-2 hydroxyl group of one glycopyranose unit can form a hydrogen bond with the C-3 hydroxyl group of the neighbouring glucopyranose unit. In the β-CD molecule a complete secondary belt is formed by these hydrogen bonds, so that β-CD is a rather rigid structure. This arrangement of hydrogen bonds can explain the observation that β-CD has the lowest solubility of all native CDs. The lower solubility of β-CD in water relative to α-and γ-CDs may also be due to the marked structure of water arising from water–β-CD interactions.
The hydrogen belt is incomplete in the α-CD molecule because one of the glycopyranose units is in a distorted position; therefore, instead of the six possible hydrogen bonds, only four can be formed. The γ-CD molecule has a more flexible structure and consequently is the most soluble of the three native CDs. The equilibrium constants for hydrogen-deuterium exchange in the secondary hydroxyl groups of α-, β- and γ-CDs also indicate that the strongest hydrogen bond system is formed in the β-CD molecule. The most important physical and chemical characteristics of native CDs can be compared (Table 1.1).
Because of their torus-like geometry, relatively hydrophobic surface of the internal cavity and the hydrophilic character of external hydroxyl groups (Figure 1.2), CD molecules easily form inclusion complexes with a wide variety of organic and inorganic molecules. This complex-forming capacity is the reason for their widespread application in chemistry and in separation science (Table 1.2).
CDs can be created with larger cavity diameters; however, these products are interesting only from a synthetic point of view, because they can contain too many water molecules, so that the driving force of complex formation is negligible.
1 Selector Properties
As has been frequently demonstrated cyclodextrins are capable of forming inclusion complexes with compounds having a size compatible with the dimensions of the cavity. Inclusion complexes are entities comprising two or more molecules; the 'host' includes a 'guest' molecule, totally or in part, by only physical forces, that is, without covalent bonding. CDs are typical host molecules and may include a great variety of molecules having the size of one or two benzene rings, or even larger compounds, which have a side chain of comparable size, to form crystalline inclusion complexes. Complexation occurs when there is a steric compatibility between the CD cavity and the guest molecule and the affinity of the guest molecule for the CD cavity is higher than for the other components present (i.e. solvent). It has been established that, besides steric compatibility, hydrophobic interactions, van der Waals interactive forces and hydrogen bonding independently or in combination play a considerable role in the determination of the strength of inclusion complexes. The stoichiometry of inclusion compounds is usually 1:1; however, complexes can be made of two or more guests (especially with the large γ-CD cavity) or with several CD molecules by the inclusion of different parts of a large guest molecule.
Many physicochemical methods have been used for the study of the formation of inclusion complexes. Thus calorimetry, spectrophotometry and various liquid chromatographic methods, such as reversed-phase thin-layer and high-performance liquid chromatography, have been successfully used for the assessment of the various aspects of host–guest interaction.
Inclusion complex formation is stereoselective; thus it affords a possibility of resolving enantiomers. A reasonable, simple three-point interaction model has been adopted for explanation of the enantiomer separations of CDs in liquid chromatography. This model is based on the assumption that one part of the host molecule, preferably an aromatic group, must interact with the cavity of the CD and the other parts of the molecule can interact with the primary and the secondary OH groups of the CD rim. As the steric matching and consequently the energy of interaction between the two enantiomers and the three points of attachment can be different, it may result in the separation of the corresponding enantiomer pair yielding enantiodifferentiation. Molecular mechanistic investigations have also been used to explain the behaviour of CDs and CD derivatives as chiral phases or chiral stationary phase additives in GC. The calculations indicated a marked opening of the rim of the primary OH groups in the cyclodextrin's cavity and of the secondary OH groups being tilted inwards to the cavity (presumably to enhance the network of secondary hydrogen bonding). It has also been deduced that the remarkable flexibility of the CD ring can be responsible for the adaptation of molecules to a wide range of shapes.
CDs have been effectively employed for chiral separations and for the improvement of a large number of separation processes in many chromatographic techniques, either as mobile-phase additives (dissolved in the mobile phase, as modifiers) or as stationary phases or stationary-phase additives. CDs offer numerous advantages. They are thermostable to a reasonable temperature which is important in GC; furthermore, they are stable over very wide pH range (2–12) and do not absorb radiation in the region normally associated with UV detection (200–350 nm) facilitating their application in liquid chromatographic techniques.
2 Solubility of Cyclodextrins
The water solubility of native cyclodextrins shows anomalous behaviour (see Table 1.1). The solubility of β-CD is only 1.85 g/100 mL at ambient temperature, whereas the solubilities of α-CD and γ-CD are significantly higher, 14.5 g/100 mL and 23.2 g/100 mL, respectively. The temperature dependences of the solubility of the three native CDs in water can be described by the following equations:
α-CD; c = (11271 [+ or -] 0.45)e-(3530[+ or -]31) [(1/T)-(1/298.1)] (1.1)
β-CD; c = (18.3236 [+ or -] 0.099)e-(14137[+ or -]31) [(1/T)-(1/298.1)] (1.2)
γ-CD; c = (219.40 [+ or -] 9.8)e-(3187[+ or -]320) [(1/T)-(1/298.1)] (1.3)
where c is the concentration of CD (mg mL-1) (the values of the constants are followed by their standard errors) and T is the temperature in Kelvin. According to Equations 1.1–3 the solubility of the CDs depends strongly on the temperature: at 50 °C the solubility of all three CDs is about three times higher than at 20 °C.
In the presence of organic molecules, the solubility of native CDs generally decreases owing to complex formation (Figure 1.3).
3 Dielectric Properties
Dielectric constants of CD cavities have been established from the change in the fluorescence properties of occluded pyrene-3-carboxaldehyde. The estimated dielectric constants of CD cavities have been calculated as 48 and 55 for β- and γ-CDs, respectively. These values can be explained by the assumption that the larger γ-CD cavity can possibly include more water molecules, thus providing a larger effective dielectric constant. The dielectric constants of native CD cavities on incorporating the toluidinyl group of 6-p-toluidinylnaphthalene-2-sulfonate at pH 5.3 and 25 °C have also been determined and were found to be 47.5, 52.0 and 70.0 for α-CD, β-CD and γ-CD, respectively. It has been further established that the dielectric constant of the CD cavity depends not only on the inner diameter of the cavity but also on the size of the included guest molecule.
4 Thermal Properties
CDs and CD derivatives have been extensively used as stationary phases or stationary-phase additives in GC. As GC analyses are generally carried out at elevated temperatures, an exact knowledge of the thermal stability of CDs and CD derivatives under the GC conditions is of paramount importance. A considerable number of thermoanalytical methods have been employed for the study of thermostability of CDs and their corresponding inclusion complexes.
The thermal degradations of native cyclodextrins and substituted β-cyclodex-trins were characterised by hot-stage microscopy (HSM), differential scanning calorimetry (DSC), thermogravimetry (TG) and X-ray powder diffractometry (XRD). The measurements indicated that cyclodextrins have no well-defined melting point, but from about 197 °C they begin to decompose. It was further found that in an inert atmosphere each of them decomposed in a single major step (250–400 °C) leaving a residue which is thermally quite stable at higher temperatures. Furthermore, it has been frequently shown that the thermal stability of CD derivatives depends on the type, location and number of any substituents. In the case of amino- and phosphate-substituted cyclodextrins, the first degradation step is the same as for native CDs, then volatile by-products are formed by oxidation below 600 °C, the residue being a ceramic-like substance stable to 800 °C. The charring process involves opening the CD rings followed by a chemical decomposition similar to that of cellulose with loss of the glucosidic structure and hydroxyl groups and a build up of carbonyl groups and aromatic structures. Carbon dioxide, water, levoglucosan and furans are the major volatiles evolved from cyclodextrin degradation in the same manner as from cellulose. Products derived from substituted CDs show that they do not simply behave as leaving groups but rather take part in the charring process.
Although chromatographic techniques mainly employ derivatised CD and not native CDs the number of studies dealing in detail with the thermal properties of CD derivatives is surprisingly low. This phenomenon is probably because CDs are used for practical purposes in chromatography (to improve separation of enantiomers and other hard to separate analytes) and the exact determination of the thermal properties of CDs has been considered as of secondary importance.
As previously mentioned CDs are not hygroscopic, but form various stable hydrates. Figure 1.4 illustrates the water sorption and desorption isotherms of native cyclodextrins.
CDs have no reducing end groups. They give positive reactions in test characteristic for non-reducing carbohydrates. Therefore, they give a colour reaction with anthrone making possible their quantitative determination by spectrophotometric methods.
Rate constants for the hydrolysis of linear and cyclic dextrins determined at various temperatures and acid concentrations have been compiled (Table 1.3). The results indicate that by decreasing the concentration of the acid at a fixed temperature, or by lowering the temperature at a fixed acid concentration, differences in the hydrolysis rates of cyclic and linear dextrins can be modified.
5 Chemically Modified Cyclodextrins (Cyclodextrin Derivatives)
In CDs every glucopyranose unit has three free OH groups, two of which (on C-2 and C-3) are secondary and one (C-6) primary. As each of these free hydroxyl groups can be modified, by substituting the hydrogen atom or the hydroxyl group by a wide variety of substituents, the majority of simple synthetic reactions results in a considerable number of positional isomers. Hydroxyl protons can be exchanged by deuterium at the oxygen–hydrogen bonds or carbon–hydrogen bonds in deuterated CD derivatives. These molecules represent the smallest group of CD derivatives and have found application only in NMR studies of CDs. Some inorganic esters of CDs, such as nitrates, sulfates, phosphates etc. have also been synthesised; however, the organic (acetyl, benzoyl, propionyl, methyl and carbamoyl) esters have been more frequently used for practical industrial and analytical purposes.
Ether derivatives are the most important CD derivatives from a practical point of view. These compounds can be prepared either by direct reaction with an alkylating agent or via an intermediate, such as sulfonate esters or deoxyhalogeno derivatives. This group equally contains neutral, anionic and cationic derivatives as well as silyl ethers.
Deoxy cyclodextrins can be classified according to the mode of preparation into two groups: intermediaries which can be further derivatised, and end products containing thio-, amino-, substituted amino- or azido-substituents.
Branched cyclodextrins (or second-generation cyclodextrins) can also be obtained by chemical synthesis but in most cases they are prepared by enzymatic reactions. Branched CDs can be divided into two categories. Homogeneous branched cyclodextrins have only glucose or malto-oligosaccharide side chains bound to the native CDs. Heterogeneous branched CDs have one or more galactose or mannose residues bonded either to each other or directly to the parent cyclodextrin rings. The solubilities of branched cyclodextrins in water, even in aqueous 80% ethanol or in aqueous 50% solutions of methanol, formaldehyde and ethylene glycol, are extremely high in comparison with their parent CDs.
It is well known that derivatisation changes the physical and chemical properties of the CDs, modifying their solubility, complex-forming capacity, thermal properties and chemical stability. Numerous CD derivatives have found application in chromatography (Table 1.4). The aim may be the improvement of the solubility of the native CDs making possible an increase in their concentration in a chromatographic mobile phases, the enhancement of the stability of the host–guest inclusion complexes by modifying the reactivity and/or mobility of the guest molecule. Furthermore, derivatisation can be employed for the formation of insoluble and/or immobilised CD-containing structures, which can be used as stationary phases for liquid and gas chromatography.
6 Cyclodextrin Polymers
These compounds consist of two or more cyclodextrin rings covalently bonded to each other using a spacer molecule. According to the synthetic pathway they can be classified into linear polymers, which are generally water soluble, and cross-linked polymers, which are water insoluble. The water soluble CD polymers have a higher solubility than their parent cyclodextrins. Many examples have been reported of the solubilising effect of cyclodextrin polymers for poorly soluble drugs.
As with native and derivatised CDs, these polymers have also found applications in chromatography. Water soluble polymers have been used as mobile phase additives in liquid chromatographic techniques and in electrically driven separations.
CHAPTER 2Use of Cyclodextrins in Gas–Liquid Chromatography
Various cyclodextrin derivatives have been used in the GC analysis of a wide range of organic compounds. The overwhelming majority of applications are for the separation of optical isomers because of the growing demands of both industrial and legislation organisations. The growing demand for pure chiral compounds and for efficient control methods have made necessary the development of GC techniques suitable for the chiral separation of a wide variety of compounds, such as pesticides, other environmental pollutants, pharmaceuticals etc. An extensive search for new synthetic routes for CD derivatives has been motivated by the relatively low thermal stability of the unmodified CDs and by the recognition that each CD derivative shows different separation characteristics but that only a limited number of chiral separations can be performed on any single CD stationary phase.
(Continues...)
Excerpted from Cyclodextrins in Chromatography by Tibor Cserháti, Esther Forgács. Copyright © 2003 The Royal Society of Chemistry. Excerpted by permission of The Royal Society of Chemistry.
All rights reserved. No part of this excerpt may be reproduced or reprinted without permission in writing from the publisher.
Excerpts are provided by Dial-A-Book Inc. solely for the personal use of visitors to this web site.
Table of Contents
Principles of Chromatography; Chemistry and Physicochemistry of Cyclodextrins; Use of Cyclodextrins in Gas-Liquid Chromatography; Supercritical Fluid Chromatography; Use of Cyclodextrins in Liquid Chromatography; Use of Cyclodextrins in Electrophoretic Techniques; Subject Index.What People are Saying About This
A good addition to all libraries of pharmaceutical and agrochemical industries and also academic institutions, drug regulatory agencies and environmental agencies.