Disturbing the Solar System: Impacts, Close Encounters, and Coming Attractions available in Paperback
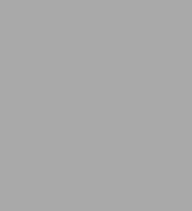
Disturbing the Solar System: Impacts, Close Encounters, and Coming Attractions
- ISBN-10:
- 0691117438
- ISBN-13:
- 9780691117430
- Pub. Date:
- 03/15/2004
- Publisher:
- Princeton University Press
- ISBN-10:
- 0691117438
- ISBN-13:
- 9780691117430
- Pub. Date:
- 03/15/2004
- Publisher:
- Princeton University Press
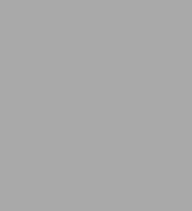
Disturbing the Solar System: Impacts, Close Encounters, and Coming Attractions
Paperback
Buy New
$44.00Buy Used
-
-
SHIP THIS ITEM
Temporarily Out of Stock Online
Please check back later for updated availability.
-
Overview
After relating a brief history of the solar system, Alan Rubin describes how astronomers determined our location in the Milky Way. He provides succinct and up-to-date accounts of the energetic interactions among planetary bodies, the generation of the Earth's magnetic field, the effects of other solar-system objects on our climate, the moon's genesis, the heating of asteroids, and the origin of the mysterious tektites. Along the way, Rubin introduces us to the individual scientistsincluding the famous, the now obscure, and the newest generation of researcherswho have enhanced our understanding of the galactic neighborhood. He shows how scientific discoveries are made; he discusses the uncertainty that presides over the boundaries of knowledge as well as the occasional reluctance of scientists to change their minds even when confronted by compelling evidence. This fresh historical perspective reveals science as it is: an imperfect but self-correcting enterprise.
Journeying to the frontiers of knowledge, Rubin concludes with the exciting realm of astrobiology. He chronicles the history of the search for life on Mars and describes cutting-edge lines of astrobiological inquiry, including panspermia (the possible transfer of life from planet to planet), the likelihood of technologically advanced alien civilizations in our galaxy, and our probable responses to alien contact.
Authoritative and up-to-date but also entertaining and fluidly written, Disturbing the Solar System will appeal to any reader who has ever picked up a rock or gazed at the moon with a sense of wonder.
Product Details
ISBN-13: | 9780691117430 |
---|---|
Publisher: | Princeton University Press |
Publication date: | 03/15/2004 |
Edition description: | New Edition |
Pages: | 384 |
Product dimensions: | 6.00(w) x 9.25(h) x (d) |
About the Author
Read an Excerpt
COPYRIGHT NOTICE: Published by Princeton University Press and copyrighted, © 2002, by Princeton University Press. All rights reserved. No part of this book may be reproduced in any form by any electronic or mechanical means (including photocopying, recording, or information storage and retrieval) without permission in writing from the publisher, except for reading and browsing via the World Wide Web. Users are not permitted to mount this file on any network servers.
Chapter 1
A BRIEF HISTORY OF THE SOLAR SYSTEM
Some people feel . . . that by delving into the inner workings of something, we destroy the essential beauty and mystery surrounding it. From my point of view, nothing could be farther from the truth . . . A rainbow is just as beautiful to someone who understands how it works as to anyone else."
James Trefil, Meditations at 10,000 Feet, 1985
THE PREHISTORY of the solar system is an astronomical saga of star birth and death, of matter collapsing into gravitationally bound clouds of gas and dust and elements being spewed into interstellar space. As the solar system formed, the story shifts to one in which gravitational perturbations and collisions between multikilometer-size objects play dominant roles.
Stars spend most of their lives in a state of balance between their tremendous gravity, which pushes matter inward, and the gas pressure caused by the energy released during nuclear fusionthe transformation of hydrogen into heliumwhich pushes matter outward (figure 1.1). But toward theend of their lives, stars run low on hydrogen and begin to release energy by creating heavier elements in their nuclear-fusion furnaces. Very massive stars can fuse helium into carbon and oxygen, and carbon into oxygen, neon, sodium and magnesium. Eventually silicon, phosphorus, and sulfur are produced and fused into iron. The fusion of heavy elements is a massive star's attempt to stave off the crushing effects of gravity, but it is a competition the star is doomed to lose. When enough iron is built up in the core, the energy spigot is turned off because it takes extra energy to split iron into lighter elements or fuse it into heavier ones.
Gravity then causes the core to collapse. As the core contracts, it heats up, eventually reaching temperatures of several billion degrees. Enormously energetic photons produced at these temperatures break down the iron nuclei into helium. This transformation uses up heat and cools the core. Gravity causes the core to collapse again. Lighter elements rain down on the core and fuse explosively into heavier ones. The massive star becomes a supernova as a shock wave blasts subatomic particles, heavy elements, and energetic photons out from the core and into the interstellar environment.
The newly formed heavy elements mix with ancient molecules that had previously escaped from the outer atmospheres of red giant stars and planetary nebulae. (These latter misnamed objects are expanding, nearly spherical clouds of hot gas ejected from old stars. They are not planets.) Much of this interstellar material becomes concentrated in giant molecular clouds such as the Orion Nebula, one of the most massive objects in the Galaxy. Giant molecular clouds are typically 300 light-years in diameter and range in mass from that of 100,000 to 1 million Suns. They are vast reservoirs of interstellar gas and dust held together by magnetic fields and turbulent motions. They contain numerous smaller dark clouds 5-15 light-years across. Gravitational instabilities, decreases in gas turbulence, leaking magnetic fields, and nearby supernova explosions can cause the small clouds to fragment, some into clumps only a few times as massive as the Sun. Loosely bound, multiple star systems known as stellar associations form from such clumps.
It seems likely that in some stellar associations, planets never formmineral grains accreting around one star may evaporate after encountering sporadic intense radiation from a large, hot neighboring star.
The cloud that formed our solar system began to rotate more rapidly as it contracted. A significant fraction of the gas, dust, and ice was compressed into a disk that planetary scientists call the solar nebula. (The planets eventually formed from this disk; this is why most planets orbit the Sun in nearly the same plane.)
The Sun's equator is presently tilted about 63 relative to the mean orbital plane of the planets around the Sun. This tilt may reflect gravitational perturbation of the nebular disk by the close passage of a star. The star itself may have been a small errant sibling of the Sun that wandered off shortly after birth. Studies of star clusters have shown that small stars tend to escape from the clusters relatively quickly. This "evaporation" process eventually leads to disintegration of small clusters.
Interstellar gas and dust fell into the solar nebula, causing it to heat up. Material flowed into the early Sun, and it eventually became so massive that gravity exerted enough pressure in the solar interior to spark nuclear fusion. Magnetic forces caused jetting of molecular gas at the poles. The narrow gas jets, which may have extended for several light-years, carried angular momentum away from the Sun. Accretion of material continued. Eventually the supply of infalling material ran out, and the jets shut off.
Early in its history, the Sun was chaotic, flaring up sporadically and depositing large amounts of energy into the nebula. (Even at middle age, the Sun is somewhat variable, exhibiting a 22-year periodicity in solar activity. The Sun is 1 percent brighter about every 11 years, near the times when the maximum number of sunspots occurs.) As the hot nebular gas around the nascent Sun cooled, silicate grains and other minerals condensed (i.e., came out of the gas phase as small solid particles). At lower temperatures, some fine-grained condensate particles aggregated into dust grains; other grains reacted with the gas to form new minerals.
The young energetic Sun generated powerful solar winds, driving water vapor and other gaseous materials out to about 5 AU, where the temperature was cold enough for water to condense into ice crystals. (An AU is an astronomical unit, equivalent to the mean distance between Earth and the Sun, about 150 million kilometers or 93 million miles.) The self-gravity of all this ice enabled an icy planet of about 10 Earth-masses to accrete quickly. This icy planet became the core of Jupiter; its large gravity field caused vast amounts of gas to be swept up from the nebula as it orbited the Sun.
At about the same time that Jupiter was forming, much else was going on in the solar nebula. Poorly understood accretionary processes were transforming dust into rocks and rocks into asteroid-size bodies known as planetesimals. Because meteorite researchers can study chips of these planetesimals and use them to infer the nature of the nebula, it is useful to recount what we think we know of how planetesimals formed.
High-energy events of some kind caused fine-grained nebular materials to evaporate, leaving behind refractory residues (materials that require high temperatures to melt or vaporize). Small chunks of rock in the primitive meteorites known as chondrites formed from these residues as well as from condensates. These chunks are rich in the refractory elements calcium, aluminum, and titanium and are known as refractory inclusions, calciumaluminum inclusions, or CAIs. Some of the CAIs were remelted.
As the nebula cooled, less-refractory materials condensed and accreted. Porous clumps of silicate-rich dust were transformed into submillimeter-size spherules of melt, possibly after having been blasted by bolts of lightning. Small molten globules of metallic iron-nickel and sulfide were expelled from the spinning molten silicate droplets by centrifugal force. The residual silicate droplets crystallized into spheroidal objects called chondrules. Some chondrules collided with others and broke apart. In many cases, whole chondrules and chondrule fragments became entrained in porous dust clumps and were melted again.
Aggregations of chondrules, chondrule fragments, calcium-aluminum inclusions, metal, sulfide, and silicate dust within the disk continued to collide with one another. On average, relative velocities were low enough that gravity eventually caused these aggregations to accrete into porous chondritic planetesimals (figure 1.2). Joining the planetesimals were small amounts of interstellar dust that had formed around dying stars and had been ejected into interstellar space; these materials accreted continuously to the nebula. As the planetesimals grew larger, their gravitational effects increased. The orbits of other planetesimals were perturbed, causing some of these bodies to collide. Such collisions caused the porous planetesimals to compress and collapse.
Most planetesimals were heated, and many were melted. A minority of researchers believe that the porous planetesimals were heated mainly by collisions. Most researchers believe that the heat came from the decay of the short-lived radionuclide aluminum 26 (i.e., aluminum atoms with 13 protons and 13 neutrons). If sufficient amounts of this isotope were available, the planetesimals would have melted. The aluminum 26 itself may have formed in a recent supernova explosion or through irradiation by the early Sun.
Minor melting along grain boundaries transformed porous materials into rocks. Many of the rocks were heated and recrystallized. In some cases, water-bearing silicate minerals that had accreted to the planetesimals were dehydrated, causing fluid alteration of materials near the surface.
All the planetesimals were hit repeatedly by meteoroids. Some regions were melted by the shock energy released by these impacts; other regions were shattered. In some cases, projectiles colliding at low relative velocities were incorporated intact into the target rocks. Fine-grained material was chipped off rocky outcrops by micrometeoroids to form unconsolidated soil. Rare-gas molecules streaming out of the Sun adhered to the surfaces of tiny mineral grains in the soil. Some grains were damaged by high-energy particles released by solar flares.
In different regions of the nebula, planetesimals colliding at low relative velocities stuck together, some eventually forming the cores of planets. The massive gravity of nascent Jupiter perturbed the orbits of nearby planetesimals, causing them to collide at higher relative velocities. Those bodies with orbital periods resonant with that of Jupiter were flushed out of the region. Many were expelled from the solar system, some fell into the Sun, and others rained down on the rocky planets in the inner solar system. The dispersal of objects in Jupiter's proximity caused a dearth of residual mass in the asteroid belt and in the vicinity of Mars. Mars ended up only 11 percent as massive as Earth.
Because the amount of material in the nebular disk in the vicinity of Uranus and Neptune was probably low, some theorists have argued that these two giant planets did not form in their present locations. Instead, their rocky cores may have formed near Jupiter and Saturn and were then gravitationally perturbed away; after a few hundred thousand years, the Uranus and Neptune embryos settled into their present orbits (figure 1.3).
As the giant planets formed, electrically conducting material flowing deep within them interacted with the magnetic field stretching out from the Sun to produce planetary magnetic fields. The conducting material was probably liquid metallic hydrogen in Jupiter and Saturn and an electrolytic solution of water in Uranus and Neptune.
As planetesimals and planetary embryos were scattered about, particularly energetic impacts of these bodies with the major planets tilted the planets and affected their rotation rates. Uranus got tipped on its side by the off-center impact of a projectile the size of Earth. The hypothesized impact on Earth more than four billion years ago of a projectile a few times the mass of Mars probably formed the Moon.
Many planetesimals collided with each other. The remnants now residing between Mars and Jupiter are known as asteroids (meaning "starlike"); these small bodies appear only as pinpoint, starlike images in all but the largest optical telescopes. Some asteroids were hit hard and disrupted, their fragments remaining in orbits similar to those of their parents. These groups of sibling objects possessing similar orbits are known as asteroid families. Other planetesimals were hit hard enough to break apart but not hard enough for most of the fragments to reach escape velocity. The fragments fell back together, forming poorly compacted, chaotic piles of rubble. This process of collisional disruption and gravitational reassembly may have affected most large asteroids, turning them inside out and mixing together materials from the cores and surfaces (figure 1.4).
Some asteroid fragments were left with distinct double-lobed shapes resembling giant peanuts or dog bones. In some cases, these asteroids may consist of two separate bodies in contact. The subsequent collisions of such objects with the Moon or Earth can produce double craters.
As the giant planets formed, small satellites accreted in orbit around them. The planets' more distant satellites were gravitationally captured as they wandered by. Even Mars (with far less mass than the giant planets) captured a couple of small asteroids in its vicinity. The gravitational fields of the planets caused most of their satellites to become tidally locked, forcing them to rotate on their axes at the same rate that they revolved around their parent planet. Hence, they always show the same face to their primary planet just as the Moon does to Earth.
Gravitational tugs on some of the moons in the outer solar system by their planet and by neighboring moons caused severe tidal heating. Their icy surfaces melted and flowed. Images of Jupiter's moon Europa taken by recent spacecraft flybys have revealed icebergs that appear to have cracked and drifted apart. Most scientists believe that Europa has an ocean of liquid water beneath a cap of ice. Its neighboring moon Io, tugged on by both Jupiter and Europa, experienced great internal heating and expelled much of its initial allotment of volatile compounds from hundreds of volcanoes dotting its surface.
Each of the giant planets produced a system of orbiting rings of dust and ice. Some rings are held in place by the gravitational pulls of small moonlets. Some rings are continuously replenished from particles knocked off nearby satellites by collisions. Some rings may have formed by collisional or tidal disruption of orbiting moons.
Asteroids occupying the same orbital plane as Jupiter but leading or trailing the planet by 603 in their orbits are in gravitationally stable locations called Lagrangian points (the so-called L4 and L5 points). Several hundred bodies known as Trojan asteroids are distributed around both of these points. Most were probably captured early in solar-system history (figure 1.5).
Icy planetesimals, commonly known as comets, accreted in the outer solar system where the giant planets now reside. Billions of these planetesimals were flung thousands of astronomical units away from the Sun by gravitational interactions with the giant planets. The Oort Cloud is the spherical swarm of these icy planetesimals at the outskirts of the solar system; it extends out to about 50,000 AU.
Other icy planetesimals remained closer to the Sun, just beyond the orbit of Neptune in a region known as the Edgeworth-Kuiper Belt. The largest of these objects is Pluto. The Edgeworth-Kuiper Belt is also the source of the vast majority of comets entering the inner solar system.
A few comets passing through the inner solar system come from the Oort Cloud. As the Sun orbits the galactic center once about every 240 million years, it occasionally passes relatively close to neighboring stars. Gravitational tugs from these stars affect the orbits of comets in the Oort Cloud. The orbits are also affected by the gravitational pull of giant molecular clouds and by the myriad stars in the galactic disk. A few of the Oort Cloud comets are diverted by these gravitational tugs toward the inner solar system. Some occasionally brighten our evening skies, and sometimes one strikes Jupiter, the Moon, or Earth, or explodes in our lower atmosphere.
After Earth had melted, devolatilized, formed a metallic-iron core, and begun to cool, the impacts of icy planetesimals brought water and other volatile compounds to the surface. Sometime around three and a half to four billion years ago, the first organisms emerged from a sterile world. As life evolved on Earth, it had to contend with volcanic eruptions, shifting continents, earthquakes, changing climates, colliding asteroids, and perhaps ionizing radiation from occasional nearby supernova explosions. Early photosynthesizing organisms produced oxygen, and within one and a half to two billion years had oxygenated the ocean and dramatically changed the composition of the atmosphere. As individual organisms lived and died, some minuscule fraction were deposited in sediments that hardened into fossil-bearing rocks. Many of these rocks were later eroded by wind, water, and moving glaciers; others were buried and metamorphosed. But many fossil-bearing rocks remained. Their study has unlocked some of the secrets of the early history of life, although life's ultimate origin has so far proven elusive.
The eventual development of consciousness and speech enabled one species to build telescopes, explore the cosmos, and ponder the possibilities of extraterrestrial life. Out of their brains poured myth, religion, mathematics, technology, philosophy, and science. It was this species, the human species, that first began telling stories of the solar system. But, as the Renaissance philosopher Francis Bacon (1561-1626) cautioned, "[t]he mind of man is like an enchanted glass, full of superstitions, apparitions, and impostures." When filtered through such an imperfect apparatus as the human mind, it is not surprising that our stories tend to reflect our cultural, political, and religious biases.
Nevertheless, our telescopes, spacecraft, and computers have provided enormous amounts of information about the present state of the solar system and its likely origin. Astronomers and solar physicists also have a good idea of the end of the story: in a few billion years, the Sun will balloon out as a red giant, engulfing Mercury and Venus and scorching the surface of Earth. The oceans will boil, and life on Earth will cease. While many details of solar-system history remain hidden, we have enough information to spin a good yarn.
A fundamental property of the solar system is its position in the Galaxy. Surprisingly perhaps, this location is important to the history of life. The center of the Galaxy is an active region, full of radiation. If the solar system had formed near there, the origin of life would have been problematic. On the other hand, stars in clusters above the plane of the Galaxy are poor in heavy elements and probably lack rocky planets. The story of how scientists deduced the location of the solar system is an instructive one in the history of astronomy. It is filled with brilliant insights, painstakingly obtained data, numerous mistakes, and agonizingly slow progress. It is the story we take up next.
Table of Contents
Preface xiPART 1: Overview of the Solar System
I. A Brief History of the Solar System 3
II. Where Are We? The Location of the Solar System 17
III. Heat Sources 33
PART 2: Earth and Moon
IV. The Magnetic Earth 47
V. Ice Ages 63
VI. Origin of the Moon 84
PART 3: Small Bodies, Impacts, and Rings
VII. Asteroids and Meteorites 99
VIII. What Heated the Asteroids? 118
IX. Mesosiderites: Biography of a Shocked and Melted Asteroid 129
X. Meteor Crater 143
XI. The Lunar Crater Controversy--a Brief Retelling 158
XII. Dinosaurs and the Cretaceous-Tertiary Extinction 167
XIII. Recent Impacts: Tunguska to Shoemaker-Levy 9 183
XIV. Tektites: A Glass Menagerie 200
XV. Rings and Shepherds 215
PART 4: Life beyond Earth
XVI. The Search for Life on Mars 235
XVII. Panspermia 259
XVIII. Paucity of Aliens 281
XIX. Human Response to First Contact 299
Epilogue 321
Glossary 325
Additional Reading 343
Index 353
What People are Saying About This
This book--which reflects Dr. Rubin's experience in writing and lecturing for public audiences--stands out for its focus, innovation, and appeal. Dr. Rubin synthesizes a great deal of modern research in geophysics and planetary science. His selection and treatment of subjects is fresh. Even when he targets more familiar material, he approaches it with an unconventional perspective.
E. C. Krupp, Director, Griffith Observatory
"This book—which reflects Dr. Rubin's experience in writing and lecturing for public audiences—stands out for its focus, innovation, and appeal. Dr. Rubin synthesizes a great deal of modern research in geophysics and planetary science. His selection and treatment of subjects is fresh. Even when he targets more familiar material, he approaches it with an unconventional perspective."—E. C. Krupp, Director, Griffith Observatory