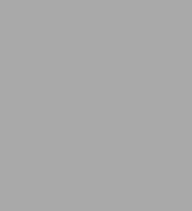
Eocene-Oligocene Climatic and Biotic Evolution
584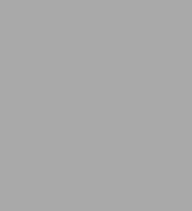
Eocene-Oligocene Climatic and Biotic Evolution
584Hardcover
-
PICK UP IN STORECheck Availability at Nearby Stores
Available within 2 business hours
Related collections and offers
Overview
Originally published in 1992.
The Princeton Legacy Library uses the latest print-on-demand technology to again make available previously out-of-print books from the distinguished backlist of Princeton University Press. These editions preserve the original texts of these important books while presenting them in durable paperback and hardcover editions. The goal of the Princeton Legacy Library is to vastly increase access to the rich scholarly heritage found in the thousands of books published by Princeton University Press since its founding in 1905.
Product Details
ISBN-13: | 9780691633954 |
---|---|
Publisher: | Princeton University Press |
Publication date: | 04/19/2016 |
Series: | Princeton Series in Geology and Paleontology , #135 |
Pages: | 584 |
Product dimensions: | 8.70(w) x 11.10(h) x 1.40(d) |
Read an Excerpt
Eocene-Oligocene Climatic and Biotic Evolution
By Donald R. Prothero, William A. Berggren
PRINCETON UNIVERSITY PRESS
Copyright © 1992 Princeton University PressAll rights reserved.
ISBN: 978-0-691-08738-2
CHAPTER 1
TOWARD A REVISED PALEOGENE GEOCHRONOLOGY
by William A. Berggren, Dennis V. Kent, John D. Obradovich, and Carl C. Swisher III
ABSTRACT
New information has become available that requires a revision of Paleogene chronology incorporated in most current Cenozoic time scales (e.g. Berggren et al., 1985a, b). Age estimates for the limits of the Paleogene (the Oligocene/Miocene and Cretaceous/Paleogene boundaries) have not changed appreciably and remain at about 24 Ma and about 66 Ma, respectively. However, new radioisotope data indicate that boundaries of subdivisions within the Paleogene are generally younger than previously estimated, for example, the Paleocene/ Eocene and Eocene/Oligocene, by about 2 to 3 m.y. We review the current status of magnetobiostratigraphic correlations and new radioisotope data, with particular reference to late Eocene—early Oligocene geochronology and provide a reassessment of the age of the Eocene/Oligocene boundary as 34 Ma. We anticipate that with concurrent work on a fundamental revision of the geomagnetic polarity sequence, a comprehensive and detailed new time scale for the Cenozoic will soon be developed.
INTRODUCTION
It is six years since we published a time scale for the Paleogene (Berggren et al., 1985a, b). All available first-order correlations between Paleogene calcareous plankton and magnetostratigraphy were compiled in Berggren et al. (1985a). Since that time there have been several additions and refinements to this data set; those relevant to late Eocene – early Oligocene geochronology are shown in Tables 1.1 (planktonic foraminifera) and 1.2 (calcareous nannoplankton). Inasmuch as we view a time scale to consist of a data set which integrates information from biostratigraphy, radioisotopes, magnetostratigraphy and sea-floor anomalies (Aubry et al., 1988), we believe that a thorough evaluation of each of these disciplines is required before any basic revision(s) can be made to the extant scale. Moreover revisions to Paleogene geochronology are but part of a more comprehensive revision to the time scale for the Cenozoic Era in which we are currently engaged. Thus we view this contribution as an interim step on the way to a fundamental revision of Cenozoic geochronology.
We review below pertinent new Paleogene radioisotopic age data with particular emphasis on those bearing on the Eocene/Oligocene boundary and provide an assessment of the current status of Paleogene geochronology.
NEW RADIOISOTOPIC DATA
A compilation of (predominantly post-1985) radioisotopic data pertinent to Paleogene geochronology is presented in Tables 1.3,1.5-1.7 and summarized and compared with the Paleogene time-scale of Berggren et al. (1985a, b) in Figure 1.1. A number of late Eocene – early Oligocene radioisotopic dates were generated from the Marche-Umbria Basin (Italy) in connection with the research for a potential Eocene/Oligocene boundary stratotype section and point (Nocchi et al., 1986; Premoli-Silva et al., 1988). We have compiled an annotated list of those dates relevant to the boundary (Table 1.3) considered reliable by the responsible authors (Montanari, 1988; Montanari et al., 1988; Odin, et al. 1988). Of particular relevance to Paleogene geochronology is the fact that the ages obtained in the northeastern Apennines are from volcanic ashes in stratigraphic sections having (in general) both magneto- and biostratigraphy. Comparison of the magnetic and biochronologically based numerical estimates for late Eocene to early Oligocene magnetic polarity chrons (Berggren et al., 1985a, b) with those based on the ages generated in the northeastern Apennines (Montanari, 1988; Montanari et al., 1988; Odin et al., 1988) (Table 1.4) reveals discrepancies between the two ranging from about 1 m.y. (Chron C9N) to 3 m.y. (Chron C16N – C17N) with younger values found in the data from the northeastern Apennines. These data suggested a need for a revision of the Eocene/Oligocene boundary (Berggren and Kent, 1988) from 36.6 Ma (Berggren et al., 1985a, b) to a value closer to 34 Ma.
Two Oligocene and one early middle Eocene calibration points were used to constrain the lower (predominantly Paleogene) part of the time scale of Berggren et al. (1985a, b). The Oligocene points were the Lone Tree Ashes J and B from Flagstaff Rim, Wyoming, dated respectively at 32.4 Ma and 34.6 Ma by Evernden et al. (1964) and correlated with Chron C12N and C13N, respectively, by Prothero et al. (1982). The supposedly long reversed magnetozone between these ashes (J and B) indicated by the K-Ar dates at Flagstaff Rim were interpreted as correlative with Chron C12R, consistent with the estimated duration of about 2.4 m.y. for Chron C12R on magnetic polarity chronologies. However, recent mean 40Ar/39Ar ages of 34.72 Ma (Ash J) and 35.97 Ma (Ash B) (Swisher and Prothero, 1990) (Table 1.5) made on single crystals of anorthoclase indicate that the stratigraphic section between these two ashes spans about half (1.25 m.y. vs. 2.2 m.y.) the time interval previously estimated based on the earlier K-Ar ages of Evernden et al. (1964), and that the paleomagnetic signature of the stratigraphic section spanning the 1.25 m.y. between these ashes (see items 6-10, Table 1.5) is to be correlated with Chron C15 (rather than Cl 2; Prothero et al., 1982; or C13 as reinterpreted by Swisher and Prothero, 1990; see Prothero and Swisher, this volume). It should be noted that Montanari (1990) also reinterpreted the magnetostratigraphy of the High Plains sections based on the assumption that the earlier radioisotopic ages were correct and assigned the reversed interval between the two ashes to Chron C13R.
In similar fashion, newly obtained 40Ar/39Ar ages on single crystals of biotite of 33.91 Ma on the Persistent White Layer (= Glory Hole Ash), just below the lower Orellan normal event and correlative with the terminal range of the titanotheres in eastern Wyoming, and of 30.58 Ma on the Upper Whitney Ash (within the upper Whitneyan normal event), suggest that the long Orellan-Whitneyan reversed interval with a duration of about 2.5 m.y. is consistent with its (re)interpretation as correlative with Chron C12R (Swisher and Prothero, 1990). The new interpretations of North American land mammal magnetobiochronology bring the terrestrial North American record more in line with the marine magnetobiochronology developed in the northern Apennines (Gubbio, Massignano), Italy (Premoli-Silva et al., 1988) and lead to a major realignment of NALM "ages" vis à vis marine chronostratigraphy (Swisher and Prothero, 1990).
There are a number of additional radioisotopic ages on Paleogene (primarily Eocene and Oligocene) stratigraphic levels, some of which have magnetostratigraphic, in addition to biostratigraphic, control (Table 1.6). We draw attention to several items of interest in the context of this paper.
1) A mean 40Ar/39Ar isochron age on basalt of 33.4 Ma on Chron C13N at Ocean Drilling Project (ODP) Site 706 (item 2, Table 1.6) should be compared with an age of 33.91 Ma on the Persistent White Layer (= Glory Hole Ash) in strata immediately below a normal polarity interval now identified as Chron C13N in Wyoming (item 5, Table 1.5).
2) Several single crystal 40Ar/39Ar ages between ~ 34 to 35 Ma in the upper part of the Yazoo Clay Formation of the Gulf Coast and within Zone NP19/20, and thus correlative with Chron C13R (items 3-5, Table 1.6 ), provide maximum ages for the Eocene/Oligocene boundary.
3) The age of 35.4 Ma on the North American tektite level at Barbados (item 6, Table 1.6) should be compared with ages of 33.9 Ma (K-Ar) and 34.4 Ma (Rb/Sr) at the base of Chron C13R in the Massignano Section (item 6, Table 1.3). The dated levels in both instances are within the Turborotalia cerroazulensis Zone, and again provide maximum ages for the Eocene/Oligocene boundary.
4) Ages of 46.2 Ma (K-Ar) and 45.7 Ma (Rb/Sr) on Zone NP15 in the lower Castle Hayne Formation (item 9, Table 1.6) of the Atlantic Coastal Plain and 46.8 Ma on the basal part of Zone NP15 and within a normal polarity interval identified as Chron C21N of Deep Sea Drilling Project (DSDP) Hole 516F (item 10, Table 1.6) support an age estimate of about 46+ Ma for Chron C21N (cf. Berggren et al., 1985a, b where Chron C21N = 48.75 to 50.34 Ma).
The early middle Eocene calibration point in the (predominantly) Paleogene part of the time scale of Berggren et al. (1985a, b) was an age estimate of 49.5 Ma for the top of Chron C21N. This was based on an interpolation from radioisotopic (K-Ar) ages on lavas and tuffs stratigraphically bracketing the top of a normal magnetozone in continental beds in the western United States correlated to a normal magnetozone in the Ardath Shale (Zone P10) in San Diego, and thence to Anomaly 21 (Flynn, 1983, a, b; 1986).
Prothero and Swisher (this volume) and work in progress by Swisher indicate that the radioisotopic dates from Wyoming used by Flynn (1986) to calibrate Chron 21N may likely be anomalously old. New 40Ar/39Ar dates on single crystals of sanidine and biotite extracted from magnetic and biostratigraphic sections in Wyoming and Texas indicate an age of 46-45 Ma for Chron C21N. The Wyoming dates used by Flynn (1986) for the correlation of Chron C21N need to be redated by single crystal methods to determine if they are anomalously old as a result of contamination from older detrital minerals.
In view of the ages of ~ 46-47 Ma for the early Chron C20R to C21N interval cited above, we regard the estimates of 49.5 Ma for the top of Chron C21N (Flynn, 1983 a, b; 1986) and the age of 49.3 Ma on ODP Site 713 basement basalt (within Zone CP13B = NP15) immediately above Anomaly 21 (item 11, Table 1.6) as anomalous in the context of this discussion.
5) The 40Ar/39Ar age of 50.5 [+ or -] 0.8 Ma on the lowest melt horizon of the Montagnais impact 38 BERGGREN, KENT, OBRADOVICH AND SWISHER structure, biostratigraphically dated as Zone NP13 and P9, late early Eocene (item 12, Table 1.6), is seen to be about 2 m.y. younger than the estimated age, 53 Ma, for this level in the time scale of Berggren et al. (1985a, b). This age is consistent with those discussed under items 4 (above) and 6 (below) indicating that the Eocene part of a revised Paleogene time scale will require an approximately 2 m.y. younger shift in values.
6) The 40Ar/39Ar plateau age of 55.1 Ma on the -17 ash of the Mo Clay sequence in Denmark (item 13, Table 1.6) provides a much needed calibration point for the early Paleogene as it lies biostratigraphically very close to the Paleocene/Eocene boundary.
7) A large number of single-crystal laser-fusion 40Ar/39Ar ages have recently been made on the Hell Creek "Z" coal and the iridium bearing (lower) Z coal of Montana (Table 1.7). The ages range essentially between ~ 66.1 and 66.3 Ma and confirm previous estimates of ~ 66 Ma (e.g., Obradovich, 1984a; Obradovich et al., 1986; chronogram in Harland et al." 1990) for the Cretaceous/Paleogene boundary.
A recent study by Izett et al. (1991) reported three laser fusion 40Ar/39Ar dates on a sample split of the Baadsgaard et al. (1988) sanidine from the Hell Creek Z-Coal. These authors reported a mean age of 64.56 [+ or -] 0.16 Ma for the sanidine, an age approximately two percent younger than that reported here. Approximately one percent of this age difference can be explained by different ages used for the irradiation monitor mineral, the international standard Minnesota hornblende MMhb-I. The age of MMhb-I was originally published by Alexander et al. (1978) with an age of 519.4 Ma. This age was used by Obradovich (1984b) who used MMhb-I as a monitor mineral for the calibration of his 40Ar/39Ar plateau age of 66.0 [+ or -] 0.54 on sanidine from the Hell Creek Z-Coal. In 1987, the age of MMhb-I was modified to 520.4 Ma by Samson and Alexander (1987). As such, Obradovich's age for the Z-Coal sanidine would be about 0.2% older or 66.1 [+ or -] 0.54 Ma. The mean 40Ar/39Ar age based on single crystals of sanidine reported in this paper of 66.12 [+ or -] 0.14 Ma on sanidine also collected from the Hell Creek Z-Coal is also based on MMhb-I as a monitor mineral with a published age of 520.4 Ma. and is remarkably consistent with the age of 66.1 [+ or -] 0.54 Ma obtained by Obradovich.
Izett et al. (1991) report a weighted mean of three total fusion 40Ar/39Ar dates of 64.56 [+ or -]0.16 Ma. This age is based on an age of 513.9 Ma for MMhb-I, an age the Menlo Park lab has recently adopted for the MMhb-I interlaboratory standard based on an extensive set of potassium and argon measurements on MMhb-I and in-house standard SB-3. What is curious is that although the 40Ar/39Ar ages reported by Izett et al. for Hell Creek Z-Coal appear consistent with the K-Ar ages reported by Baadsgaard et al. (1988), they are based on an age of 513.9 Ma for MMhb-I (Izett et al., 1991) while the Australian National University (ANU) reports an age of 524.2 Ma for MMhb-I (Baadsgaard et al., 1988). If an age of 520.4 Ma is used for the Minnesota standard, the Menlo Park results become 65.4 Ma, an age still approximately 1 % younger than the ages adopted here. Obviously, the age of MMhb-I has not been fully resolved at the present time. As a result, we have chosen to report our ages here based on the published age of MMhb-I of 520.4 Ma as this age is most consistent with other data used in the calibration of the current time scale of Berggren et al. (1985a, b). If a younger or even older age for MMhb-I is adopted in the future, then all time scale calibration points will have to be evaluated together, not just those at the K/P boundary.
The other percent age difference for the K/P boundary is not as easily explained. Work in progress indicates that it may also be a bias in the absolute measurement of the 40Ar/39Ar of the standard. In one interlaboratory experiment, similar raw 40Ar/39Ar ratios were obtained on the K/P sanidine, but about a percent difference for the standard Fish Canyon whose age is based on K-Ar dates and, in part, on the age of MMhb-I. The actual cause of this discrepancy has not been agreed upon at this time, but work in progress will hopefully resolve this issue. We have chosen at this time to continue to use an age of approximately 66 Ma for the K/P boundary until this interlaboratory bias is resolved.
(Continues...)
Excerpted from Eocene-Oligocene Climatic and Biotic Evolution by Donald R. Prothero, William A. Berggren. Copyright © 1992 Princeton University Press. Excerpted by permission of PRINCETON UNIVERSITY PRESS.
All rights reserved. No part of this excerpt may be reproduced or reprinted without permission in writing from the publisher.
Excerpts are provided by Dial-A-Book Inc. solely for the personal use of visitors to this web site.
Table of Contents
List of Contributors
Preface
Eocene-Oligocene Climatic and Biotic Evolution: An Overview 1
Pt. I The Chronological Framework
1 Toward a Revised Paleogene Geochronology 29
2 Magnetostratigraphy and Geochronology of the Terrestrial Eocene-Oligocene Transition in North America 46
3 Magnetostratigraphy of the Lower and Middle Members of the Devil's Graveyard Formation (Middle Eocene), Trans-Pecos Texas 74
4 Redefinition of the Duchesnean Land Mammal "Age," Late Eocene of Western North America 88
5 Mammalian Range Zones in the Chadronian White River Formation at Flagstaff Rim, Wyoming 106
6 Eocene-Oligocene Climatic Change in North America: The White River Formation near Douglas East-Central Wyoming 116
Pt. II Climatic Events
7 Evidence from the Antarctic Continental Margin of Late Paleogene Ice Sheets: A Manifestation of Plate Reorganization and Synchronous Changes in Atmospheric Circulation over the Emerging Southern Ocean? 131
8 Middle Eocene to Oligocene Stable Isotopes Climate, and Deep-Water History: The Terminal Eocene Event? 160
9 Late Eocene and Early Oligocene in Southern Australia: Local Neritic Signals of Global Oceanic Changes 178
10 Paleogene Climatic Evolution: A Climate Model Investigation of the Influence of Continental Elevation and Sea-Surface Temperature upon Continental Climate 202
Pt. III The Marine Record
11 Eocene-Oligocene Faunal Turnover in Planktic Foraminifera, and Antarctic Glaciation 218
12 Middle Eocene-Late Oligocene Bathyal Benthic Foraminifera (Weddell Sea): Faunal Changes and Implications for Ocean Circulation 245
13 Late Paleogene Calcareous Nannoplankton Evolution: A Tale of Climatic Deterioration 272
14 Middle Eocene through Early Miocene Diatom Floral Turnover 310
15 Late Paleogene Dinoflagellate Cysts with Special Reference to the Eocene/Oligocene Boundary 327
16 The Patterns and Causes of Molluscan Extinction across the Eocene/Oligocene Boundary 341
17 Evolution of Paleogene Echinoids: A Global and Regional View 349
18 Cetacean Evolution and Eocene/Oligocene Environments 368
Pt. IV The Terrestrial Record
19 Paleosols and Changes in Climate and Vegetation across the Eocene/Oligocene Boundary 382
20 Low-Biomass Vegetation in the Oligocene? 399
21 Climatic, Floristic, and Vegetational Changes near the Eocene/Oligocene Boundary in North America 421
22 Vegetational and Floristic Changes around the Eocene/Oligocene Boundary in Western and Central Europe 437
23 Western North American Reptile and Amphibian Record across the Eocene/ Oligocene Boundary and Its Climatic Implications 451
24 Mammalian Faunas in North America of Bridgerian to Early Ankareean "Ages" (Eocene and Oligocene) 464
25 British Mammalian Paleocommunities across the Eocene-Oligocene Transition and Their Environmental Implications 494
26 The Evolution of Mammalian Faunas in Europe during the Eocene and Oligocene 516
27 The Chinese Oligocene: A Preliminary Review of Mammalian Localities and Local Faunas 529
28 The Eocene-Oligocene Transition in Continental Africa 548
Index 567