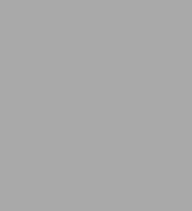
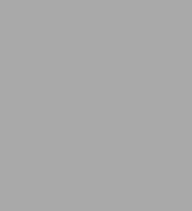
eBook
Available on Compatible NOOK devices, the free NOOK App and in My Digital Library.
Related collections and offers
Overview
Since the late 1960s the Internet has grown from a single experimental network serving a dozen sites in the United States to a network of networks linking millions of computers worldwide. In Inventing the Internet, Janet Abbate recounts the key players and technologies that allowed the Internet to develop; but her main focus is always on the social and cultural factors that influenced the Internets design and use. The story she unfolds is an often twisting tale of collaboration and conflict among a remarkable variety of players, including government and military agencies, computer scientists in academia and industry, graduate students, telecommunications companies, standards organizations, and network users.
The story starts with the early networking breakthroughs formulated in Cold War think tanks and realized in the Defense Department's creation of the ARPANET. It ends with the emergence of the Internet and its rapid and seemingly chaotic growth. Abbate looks at how academic and military influences and attitudes shaped both networks; how the usual lines between producer and user of a technology were crossed with interesting and unique results; and how later users invented their own very successful applications, such as electronic mail and the World Wide Web. She concludes that such applications continue the trend of decentralized, user-driven development that has characterized the Internet's entire history and that the key to the Internet's success has been a commitment to flexibility and diversity, both in technical design and in organizational culture.
Product Details
ISBN-13: | 9780262261333 |
---|---|
Publisher: | MIT Press |
Publication date: | 07/24/2000 |
Series: | Inside Technology |
Sold by: | Penguin Random House Publisher Services |
Format: | eBook |
Pages: | 268 |
File size: | 1 MB |
About the Author
Read an Excerpt
Chapter One
White Heat and Cold War: The Origins
and Meanings of Packet Switching
Of all the ARPANET's technical innovations, perhaps the most celebrated was packet switching. Packet switching was an experimental, even controversial method for transmitting data across a network. Its proponents claimed that it would increase the efficiency, reliability, and speed of data communications, but it was also quite complex to implement, and some communications experts argued that the technique would never work. Indeed, one reason the ARPANET became the focus of so much attention within the computer science community was that it represented the first large-scale demonstration of the feasibility of packet switching. The successful use of packet switching in the ARPANET and in other early networks paved the way for the technique's widespread adoption, and at the end of the twentieth century packet switching continued to be the dominant networking practice. It had moved from the margins to the center, from experimental to "normal" technology.
Many computer professionals have seen packet switching as having obvious technical advantages over alternative methods for transmitting data, and they have tended to treat its widespread adoption as a natural result of these advantages. In fact, however, the success of packet switching was not a sure thing, and for many years there was no consensus on what its defining characteristics were, what advantages it offered, or how it should be implementedin part because computer scientists evaluated it in ideological as well as technical terms.Before packet switching could achieve legitimacy in the eyes of data communications practitioners, its proponents had to prove that it would work by building demonstration networks. The wide disparity in the outcomes of these early experiments with packet switching demonstrates that the concept could be realized in very different ways, and that, far from being a straightforward matter of a superior technology's winning out, the "success" of packet switching depended greatly on how it was interpreted.
Packet switching was invented independently by two computer researchers working in very different contexts: Paul Baran at the Rand Corporation in the United States and Donald Davies at the National Physical Laboratory in England. Baran was first to explore the idea, around 1960; Davies came up with his own version of packet switching a few years later and subsequently learned of Baran's prior work. Davies was instrumental in passing on the knowledge of packet switching that he and Baran had developed to Lawrence Roberts, who was in charge of creating the ARPANET. This chain of invention and dissemination has become a standard element of origin stories about the Internet; indeed, it is easy to get the impression that packet switching simply took a detour through the United Kingdom before re-emerging, unchanged, in the United States to fulfill its destiny as the underlying technology of the ARPANET.
However, while Baran's and Davies's versions of packet switching had some basic technical similarities, their conceptions of what defined packet switching and of what it was good for were very different. Much of this difference was due to the strong political pressures that were brought to bear on computing research in the United Kingdom and in the United States. Large computer projects in both countries were developed in a context of government funding and control, and national leaders saw computers as a strategic technology for achieving important political goals. But in the very different policy contexts of the United States and the United Kingdom, packet switching took on different meanings for Baran, Davies, and Roberts. Packet switching was never adopted on the basis of purely technical criteria, but always because it fit into a broader socio-technical understanding of how data networks could and should be used.
Networking Dr. Strangelove: The Cold War Roots of Packet Switching in the United States
As the 1960s opened, relations between the United States and the Union of Soviet Socialist Republics were distinctly chilly. The USSR had launched its Sputnik satellite in 1957, setting off alarm in the United States over a "science gap" and prompting a surge of government investment in science and technology. A series of events kept the Cold War in the public consciousness: an American U-2 spy plane was shot down over the USSR in 1960, the Berlin Wall went up in 1961, and 1962 brought the Cuban Missile Crisis. The shadow of nuclear war loomed over popular culture. The novels On the Beach (Shute 1957) and Fail-Safe (Burdick and Wheeler 1962)both made into movies in the early 1960spresented chilling accounts of nuclear war and its aftermath. And in 1964, movie theaters across the United States presented a brilliant black comedy of Cold War paranoia, Dr. Strangelove (Kubrick 1963).
Dr. Strangelove, though humorous, highlighted the vulnerability of the United States' communications channels to disruption by a Soviet attack, which might make them unavailable just when they were needed most. In the movie, a psychotic Air Force commander named Jack D. Ripper sets a nuclear holocaust in motion by invoking a strategy of mutual assured destruction called "Plan R." This planwhich allows Ripper to circumvent the president's authority to declare waris specifically designed to compensate for a wartime failure in command, control, and communications. In the movie, an Air Force general explains:
Plan R is an emergency war plan in which a lower-echelon commander may order nuclear retaliation after a sneak attackif the normal chain of command has been disrupted.... The idea was to discourage the Russkies from any hope that they could knock out Washington ... as part of a general sneak attack and escape retaliation because of lack of proper command and control.
Plan R allows Ripper to launch a "retaliatory" attack even though no first strike has actually occurred. In reality (as the film's disclaimer states), the US Air Force never had any such strategy. Even before Dr. Strangelove opened, the Air Force was exploring a very different solution to the threat of a first strike: building a communications system that would be able to survive an attack and so that "proper command and control" could be maintained. As Edwards (1996, p. 133) has documented, Cold War defense analysts saw robust communications networks as a necessity in any nuclear confrontation: "Flexible-response strategy required that political leaders continue to communicate during an escalating nuclear exchange.... Therefore, preserving central command and controlpolitical leadership, but also reconnaissance, data, and communications linksachieved the highest military priority." The need for "survivable communications" was generally recognized by the early 1960s. Among those intent on filling it was a researcher at the Air Force's premier "think tank," the Rand Corporation.
Founded by the Air Force in 1946 as an outgrowth of operations research efforts initiated during World War II, Rand (originally RAND, derived from "research and development") was a nonprofit corporation dedicated to research on military strategy and technology. Rand was primarily funded by contracts from the Air Force, though it served other government agencies as well. It attracted talented minds though a combination of high salaries, relative autonomy for researchers, and the chance to contribute to policy decisions of the highest importance (Baran 1990, pp. 10, 11). Edwards (1996, p. 116) notes that "Rand was the center of civilian intellectual involvement in defense problems of the 1950s, especially the overarching issue of nuclear politics and strategy." Rand's role was visible enough to be reflected in popular culturefor example, the fictional Dr. Strangelove turns to "the Bland Corporation" when he needs advice on nuclear strategy. Because its approach to systems analysis emphasized quantitative models and simulation, Rand was also active in computer science research (Edwards 1996, pp. 122-124).
In 1959 a young engineer named Paul Baran joined Rand's computer science department. Immersed in a corporate culture focused on the Cold War, Baran soon developed an interest in survivable communications, which he felt would decrease the temptation of military leaders to launch a preemptive first strike:
Both the US and USSR were building hair-trigger nuclear ballistic missile systems.... If the strategic weapons command and control systems could be more survivable, then the country's retaliatory capability could better allow it to withstand an attack and still function; a more stable position. But this was not a wholly feasible concept, because long-distance communications networks at that time were extremely vulnerable and not able to survive attack. That was the issue. Here a most dangerous situation was created by the lack of a survivable communication system. (Baran 1990, p. 11)
Baran was able to explore this idea without an explicit contract from the Air Force (ibid., pp. 12, 16), since Rand had a considerable amount of open-ended funding that researchers could use to pursue projects they deemed relevant to the United States' defense concerns.
Baran began in 1959 with a plan for a minimal communications system that could transmit a simple "Go/No go" message from the president to commanders by means of AM radio. When Baran presented this idea to military officers, they immediately insisted that they needed greater communications capacity. Baran spent the next three years formulating ideas for a new communications system that would combine survivability with high capacity (ibid., pp. 14-15). He envisioned a system would allow military personnel to carry on voice conversations or to use teletype, facsimile, or low-speed computer terminals under wartime conditions. The key to this new system was a technique that Baran (1960, p. 3) called "distributed communications." In a conventional communications system, such as the telephone network, switching is concentrated and hierarchical. Calls go first to a local office, then to a regional or national switching office if a connection beyond the local area is needed. Each user is connected to only one local office, and each local office serves a large number of users. Thus, destroying a single local office would cut off many users from the network. A distributed system would have many switching nodes, and many links attached to each node. The redundancy would make it harder to cut off service to users.
In Baran's proposed system, each of several hundred switching nodes would be connected to other nodes by as many as eight lines (figure 1.1). Several hundred multiplexing stations would provide an interface between the users and the network. Each multiplexing station would be connected to two or three switching nodes and to as many as 1024 users with data terminals or digital telephones. The switching was distributed among all the nodes in the network, so knocking out a few important centers would not disable the whole network. To make the system even more secure, Baran (1964a, volume VIII, section V) planned to locate the nodes far from population centers (which were considered military targets), and he designed the multiplexing stations with a wide margin of excess capacity (on the assumption that attacks would cause some equipment to fail). Baran added such military features as cryptography and a priority system that would allow high-level users to preempt messages from lower-level users.
To move data through the network, Baran adapted a technique known as "message switching" or "store-and-forward switching." A common example of message switching is the postal system. In a message switching system, each message (e.g., a letter) is labeled with its origin and its destination and is then passed from node to node through the network. A message is temporarily stored at each node (e.g., a post office) until it can be forwarded to the next node or the final destination. Each successive node uses the address information to determine the next step of the route. In the 1930s, message switching came into use in telegraphy: a message was stored on paper tape at each intermediate station before being transmitted to the next station. At first, telegraph messages were switched manually by the telegraph operators; however, in the 1960s telegraph offices began to use computers to store and route the messages (Campbell-Kelly 1988, p. 224).
For the postal and telegraph systems, message switching was more efficient than transmitting messages or letters directly from a source to a destination. Letters are stored temporarily at a post office so that a large number can be gathered for each delivery route. In telegraphy, message switching also addressed the uneven flow of traffic on the expensive long-distance lines. In periods of light traffic, excess capacity was wasted; when the lines were overloaded, there was a risk that some messages would be lost. Storing messages at intermediate stations made it possible to even out the flow: if a line was busy, messages could be stored at the switch until the line was free. In this way, message switching increased the efficiency, and hence the economy, of long-distance telegraphy.
Besides appreciating the efficiency offered by message switching, Baran saw it as a way to make his system more survivable. Since the nodes in a message switching system act independently in processing the messages and there are no preset routes between nodes, the nodes can adapt to changing conditions by picking the route that is best at any moment. Baran (1964b, p. 8) described it this way: "There is no central control; only a simple local routing policy is performed at each node, yet the over-all system adapts." This increases the ability of the system to survive an attack, since the nodes can reroute messages around non-functioning parts of the network. Baran realized that survivability depended on more than just having redundant links; the nodes must be able to make use of those extra links. "Survivability," Baran wrote (1964a, volume V, section I), "is a function of switching flexibility." Therefore, his network design was characterized by distributed routing as well as distributed links.
Departures from Other Contemporary Systems
Paul Baran was not the first to propose either message switching or survivable communications to the military. Systems of both types already existed or were in development. A look at the state of the art in these areas makes it easier to see what aspects of Baran's ideas were really innovative and why he saw opportunities to depart from contemporary practice in certain areas.
Message switching systems were nothing new to the Department of Defense, but the existing systems were cumbersome and inefficient. Baran discovered this when he served as a member of a Department of Defense committee charged with examining several existing or proposed store-and-forward data systems in the early 1960s. These systems had such low capacity that backlogs of messages tended to build up at the switches. Therefore, the switches had to be built with large storage capacity to hold the messages that were waiting to be forwarded, and the switching computers ended up being large and complex. Baran was convinced that a network could and should be built using much higher transmission speeds, eliminating the bottlenecks at the nodes. Besides the obvious benefit of getting messages delivered faster, a high-speed, low-storage system could have switching nodes that were much simpler and cheaper than those used in contemporary store-and-forward data systems. As Baran (1964b, p. 6) pointed out, although the high-speed system would be store-and-forward in its design, in practice messages would spend little time being stored at the nodes; to the user, therefore, a connection would seem to be real-time. Baran's argument (1990, p. 24) that it was possible to build a message switching network with fast end-to-end transmission of messages and small, inexpensive switches was a radical challenge to the existing understanding of such systems.
The concept of "distributed communications" (or "distributed networks") also predated Baran; indeed, his publications cite examples of the idea from mathematics, artificial intelligence, and civilian and military communications (Baran 1964a, volume V, section I). In particular, military planners had already proposed a variety of systems based on a network of decentralized nodes linked by multiple connections (ibid., section IV). Though they shared the idea of distributed communications, however, these other systems differed in essential ways from Baran's proposal. In particular, most of them seem to have entailed the use of simple broadcast techniques, with every message going to every destination, whereas Baran's system would route messages individually through the network.
Most of the distributed systems Baran described were only proposals, not working systems. However, there was one large distributed communications network under actual development in the early 1960s. This was AUTOVON, designed and operated for the Department of Defense by the American Telephone and Telegraph Corporation. In 1961 AT&T had provided the Army with a communications network called the Switched Circuit Automatic Network, and in 1963 the corporation provided a similar network for the Air Force called North American Air Defense Command/Automatic Dial Switching. The Defense Communications Agency, which was charged with coordinating the provision of communications services throughout the armed services, decided to integrate these networks into a new system called the Continental United States Automatic Voice Network (CONUS AUTOVON). AUTOVON was not a message switching system; it was a special military voice network built on top of the existing civilian telephone network. It went into service with ten switching nodes in April of 1964 (Schindler 1982, pp. 266-269).
Describing the AUTOVON system, AT&T's magazine Long Lines (1965, p. 3) noted: "The top requirement is that the system can survive disaster." Survivability was sought in part by placing the switching centers in "hardened" sites, often underground, away from major metropolitan targets. The main survivability feature, however, was that the network was arranged in what AT&T called a "polygrid," with each switch connected to several links and with the links distributed evenly throughout the system (rather than having all connections routed through a few central switches). AT&T's publicity stressed that this redundant, decentralized system represented a sharp departure from the hierarchical structure used in the ordinary toll network. AUTOVON had one node for every few hundred lines, whereas in the regular toll system a node typically served a few thousand lines. "The polygrid network," according to the system's architects, "plays a major role in the survivability of AUTOVON. Along with its other virtues of flexibility and economy, polygrid represents the best method that technology can now offer for the rapid and reliable connection of defense communications." (Gorgas 1968, p. 227)
Baran's approach differed from AT&T's in two significant ways. First, although AUTOVON had nodes distributed throughout the system, control of those nodes was concentrated in a single operations center, where operators monitored warning lights, analyzed traffic levels, and controlled system operations. If traffic had to be rerouted, it was done manually: operators at the control center would make the decision and then contact the operators at the switching nodes with instructions to change routes (Gorgas 1968, p. 223; Long Lines 1969). In Baran's network, control was fully distributed, as noted above. Nodes would be individually responsible for determining routes, and would do so automatically without human intervention: "The intelligence required to switch signals to surviving links is at the link nodes and not at one or a few centralized switching centers." (Baran 1960, p. 3) Clearly such a system would be more survivable than one dependent on a single operations centerwhich, Baran noted, "forms a single, very attractive target in the thermonuclear era" (1964a, volume V, section II).
One implication of Baran's design was that the nodes would have to have enough "intelligence" to perform their own routingthey would have to be computers, not just telephone switches. This brings us to Baran's second departure from the AT&T approach: Baran envisioned an all-digital network, with computerized switches and digital transmission across the links. The complexity of routing messages would require computers at the nodes, since the switches would have to be able to determine, on their own, the best path to any destination, and to update that information as network conditions changed. Such computerized switches had never been designed before. "These problems," Baran acknowledged (1964b, p. 6), "place difficult requirements on the switching. However, the development of digital computer technology has advanced so rapidly that it now appears possible to satisfy these requirements by a moderate amount of digital equipment." Preserving the clarity of the signal would require that transmission be digital as well. One consequence of having a distributed network was that a connection between any two endpoints would typically be made up of many short links rather than a few long ones, with messages passing through many nodes on the way to their destinations. Having many links in a route was problematic for the transmission of ordinary analog signals: the signal degenerated slightly whenever it was switched from one link to another, and distortion accumulated with each additional link. Digital signals, on the other hand, could be regenerated at each switch; thus, digital transmission would allow the use of many links without cumulative distortion and errors. Digital transmission was still a novelty at the time; Bell Labs had only begun developing its T1 digital trunk lines in 1955, and they would not be ready for commercial service in the Bell System until 1962 (O'Neill 1985).
Baran's system would push contemporary switching and transmission technology to their limits, so it is understandable that contemporary experts reacted skeptically to his claims. The engineers in AT&T's Long Lines Division, which ran the long-distance telephone service and the AUTOVON system, tended to be familiar only with analog technology, and they doubted Baran's claims that an all-digital system could transcend the well-known limits on the number of links per call (Baran 1990, p. 18). Whereas in AUTOVON there was a maximum of seven links in any route, Baran's simulations of network routing in a small version of his system showed as many as 23 links between endpoints (Gorgas 1968, 223; Baran 1964b, p. 7, figure 11). Evidently, Baran's position outside the community of analog communications practitioners and his awareness of the potential of computer techniques made it easier for him to question the accepted limits. He had no stake in analog telephony, and his training and background in computing made it easier for him to envision an all-digital system as a way of achieving his goal of distributed communication.
And Baran's system departed from traditional telephone company practice in other ways that show the effect of Cold War military considerations on his design assumptions. For instance, AT&T tried to increase the reliability of the phone system as a whole by making each component as reliable as possible, and for an additional fee would provide lines that were specially conditioned to have lower error rates. Baran chose instead to make do with lower-quality communications links and to provide redundant components to compensate for failures. Conditioned lines would be too expensive for a system with so many links, and in any case the reliability of individual components could not be counted on in wartime conditions. "Reliability and raw error rates are secondary," observed Baran (1964b, pp. 4-5). "The network must be built with the expectation of heavy damage anyway."
Packet Switching in Baran's System
Baran's proposed network began as a distributed message switching system. His final innovation was to alter message switching to create a new technique: packet switching. In his system a message could be anything from digitized speech to computer data, but the fact that these messages were all sent in digital formas a series of binary numbers ("bits")meant that the information could be manipulated in new ways. Baran proposed that, rather than sending messages of varying sizes across the network, messages should be divided into fixed-size units that he called "message blocks." The multiplexing stations that connected users to the network would be responsible for dividing outgoing messages into uniform blocks of 1024 bits. A short message could be sent as a single block; longer messages would require multiple message blocks. The multiplexer would add to each block a header specifying the addresses of the sending and receiving parties as well as other control information. The switching nodes would use the header information to determine what route each block should take to its destination; since each block was routed independently, the different blocks that made up a single message might be sent on different routes. When the blocks reached their destination, the local multiplexer would strip the header information from each block and reassemble the blocks to form the complete message. This idea would eventually be widely adopted for use in computer networks; the message blocks would come to be called "packets" and the technique "packet switching."
For all its eventual significance, the decision to transmit data as packets was not the original focus of Baran's work. As the title of his eleven-volume work On Distributed Communications indicates, Baran began with the idea of building a distributed networkan idea that had already been identified with survivability by people working in military communications (Baran 1964a, volume V). In describing the system, Baran tended to stress the idea of link redundancy, rather than other elements such as packet switching. But as he developed the details of the system, the use of message blocks emerged as a fundamental element. By the time he wrote the final volume of the series, Baran had changed the name he used to refer to the system to reflect the new emphasis: "While preparing the draft of this concluding number, it became evident that a distinct and specific system was being described, which we have now chosen to call the 'Distributed Adaptive Message Block Network,' in order to distinguish it from the growing set of other distributed networks and systems." (Baran 1964a, volume XI, section I) What, then, was so important about packet switching? What did it mean to Baran and his sponsors?
Transmitting packets rather than complete messages imposed certain costs on the system. The interface computers had to perform the work of dividing users' outgoing messages into packets and of reassembling incoming packets into messages. There was also the overhead of having to include address and control information with each packet (rather than once per message), which increased the amount of data that had to be transmitted over the network. And since packets from a single message could take different routes to their destination, they might arrive out of sequence, which meant that there had to be provisions for reassembling them in the proper order. All this made the system more complex and presented more opportunities for failure. For Baran, these costs were outweighed by his belief that packet switching would support some of the fundamental goals of the system.
Packet switching offered a variety of benefits. Baran was determined to use small, inexpensive computers for his system, rather than the huge ones he had seen in other message switching systems, and he was aware that the switching computers would have to be simple in order to be both fast and inexpensive. The use of fixed-size packets rather than variable-size messages could simplify the design of the switching node. Another advantage for the military was that breaking messages into packets and sending them along different routes to their destination would make it harder for spies to eavesdrop on a conversation. But the biggest potential reward was efficient and flexible transmission of data. "Most importantly," wrote Baran (1964b, p. 6), "standardized data blocks permit many simultaneous users, each with widely different bandwidth requirements[,] to economically share a broad-band network made up of varied data rate links." In other words, packet switching allowed a more efficient form of multiplexing (sharing of a single communication channel by many users).
In the conventional telecommunications systems of the early 1960s, the usual form of multiplexing was by frequency division: each caller would be assigned a particular frequency band for their exclusive use for the duration of their connection. If the caller did not talk or send data continuously, the idle time would be wasted. In an alternative method, called "time division multiplexing," time is divided into short intervals, and each user in turn is given a chance to transmit data for the duration of one interval. Only users with data to transmit are offered time slots, so no slots go idle as long as anyone has data to transmit; this makes time division multiplexing more efficient for usage situations where bursts of information alternate with idle periods. Since computer data tends to have this "bursty" characteristic, Baran (1964b, p. 6) felt that time division was a more "natural" form of multiplexing for data transmission. And since the time slot accommodated a fixed amount of data, Baran believed that the use of fixed-size message blocks was a prerequisite for time division multiplexing. Thus, he associated packet switching with time division multiplexing and its promise of efficient data transmission.
Packet switching would also make it easier to combine links having different data rates in the network. The data rate is the number of bits per second that can be transmitted on a given link. In the conventional telephone system, each caller is connected at a fixed data rate, and data must flow into and out of a switch at predetermined rates. With packet switching, data flowing into a switch can be divided among the outgoing links in a variety of ways, rather than having to be sent out at a fixed rate. This would make it easier for devices transmitting data at different rates (computers and digital telephones, for instance) to share a link to the network. The system could also take advantage of new media, such as low-cost microwave transmission, that had different data rates than the standard phone company circuits. Though packet switching made the system more complex in some respects, in other ways it made the system simpler and less costly to build.
In sum, packet switching appealed to Baran because it seemed to meet the requirements of a survivable military system. Cheaper nodes and links made it economically feasible to build a highly redundant (and therefore robust) network. Efficient transmission made it possible for commanders to have the higher communications capacity they wanted. Dividing messages into packets increased security by making it harder to intercept intelligible messages. Packet switching, as Baran understood it, made perfect sense in the Cold War context of his proposed system.
The Impact of Baran's Work
For a brief time after its publication in 1964, it seemed that Baran's On Distributed Communications might soon become the blueprint for a nationwide distributed packet switching network. In August of 1965, Rand officially recommended that the Air Force proceed with research and development on a "distributed adaptive message-block network." Enthusiastic about the proposal, Air Force representatives sent it for review to the Defense Communications Agency, which oversaw the provision of military communications services (Baran 1990, Attachment 2). The DCA was one of many agencies that had been created in an attempt to bring military operations under the central control of the Department of Defense rather than allowing each of the armed services to build its own systems. In accordance with this centralizing strategy, the DoD administration made it clear during the review process that any new network would be built not by Air Force contractors but by the DCA, which had no expertise in digital technology. Baran and his Air Force sponsors, doubting that the DCA would be able to build the system that Baran had described, reluctantly decided to scrap the proposal rather than risk having it executed badly, which would waste large sums of money and perhaps discredit Baran's ideas (Baran 1990, pp. 33-35).
Though the proposed network was never built, Baran's ideas were widely disseminated among researchers interested in new communications technologies. Following Rand's standard practice, Baran presented his work to various outside experts for comment as he was developing his ideas. Eleven volumes of reports published in 1964 were widely distributed to individuals, government agencies, Rand depository libraries, and other people working in the field. The first volume was also published as an article in the March 1964 issue of IEEE Transactions on Communications Systems, and an abstract appeared in the August 1964 issue of IEEE Spectrum (a magazine for electrical and computing engineers with an estimated circulation of 160,000). Baran also lectured on his work at various universities (Baran 1990, pp. 32-33, 36). It is not clear how many researchers were immediately influenced by Baran's ideas through these channels. Most academic computer scientists were not concerned with the survivability of communications, and they may not have seen the applicability of Baran's research to their own interests. Several years later, however, his work would begin to receive wide attention as one of the technical foundations of the ARPANET. Curiously enough, the connection between these two American networking efforts would be made via a laboratory in England.
(Continues...)
What People are Saying About This
This sophisticated history is the best account so far published of the unpredictable and turbulent evolution of the Internet. With its broad international context, the book will be of value to makers and users of the global communications network, as well as to science and technology policy makers.
This sophisticated history is the best account so far published of the unpredictable and turbulent evolution of the Internet. With its broad international context, the book will be of value to makers and users of the global communications network, as well as to science and technology policy makers.
Martin Campbell-Kelly, Reader in Computer Science, University of Warwick, UK
This sophisticated history is the best account so far published of the unpredictable and turbulent evolution of the Internet. With its broad international context, the book will be of value to makers and users of the global communications network, as well as to science and technology policy makers.
Martin Campbell-Kelly, Reader in Computer Science, University of Warwick, UK