Forewords by:
Sidney Altman, Yale University, Winner of the Nobel Prize in Chemistry, 1989
and
Victor R. Ambros, Dartmouth Medical School, Co-discoverer of MicroRNAs
Forewords by:
Sidney Altman, Yale University, Winner of the Nobel Prize in Chemistry, 1989
and
Victor R. Ambros, Dartmouth Medical School, Co-discoverer of MicroRNAs
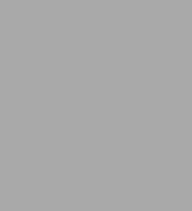
MicroRNAs: From Basic Science to Disease Biology
580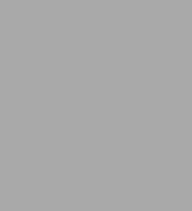
MicroRNAs: From Basic Science to Disease Biology
580Paperback(New Edition)
-
SHIP THIS ITEMIn stock. Ships in 1-2 days.PICK UP IN STORE
Your local store may have stock of this item.
Available within 2 business hours
Related collections and offers
Overview
Forewords by:
Sidney Altman, Yale University, Winner of the Nobel Prize in Chemistry, 1989
and
Victor R. Ambros, Dartmouth Medical School, Co-discoverer of MicroRNAs
Product Details
ISBN-13: | 9780521118552 |
---|---|
Publisher: | Cambridge University Press |
Publication date: | 08/20/2009 |
Edition description: | New Edition |
Pages: | 580 |
Product dimensions: | 7.00(w) x 10.00(h) x 1.40(d) |
About the Author
Krishnarao Appasani is the Founder and Chief Executive Officer of Gene Expression Systems, a gene discovery company, focusing on functional genomics in cancer research. He is the Editor of RNA Interference: From Basic Science to Drug Development (2005).
Read an Excerpt
Cambridge University Press
9780521865982 - MicroRNAs - From Basic Science to Disease Biology - Edited by Krishnarao Appasani
Excerpt
Introduction
Krishnarao Appasani
It is now clear an extensive miRNA world was flying almost unseen by genetic radar.
Gary Ruvkun, Professor, Harvard Medical School; Cell, S116, S95, 2004
RNomics is a newly emerging sub-discipline of genomics that categorically studies the structure, function and processes of non-coding ribo nucleic acids in a cell. MicroRNomics is a sub-field of RNomics that describes the biogenesis and mechanisms of tiny RNA regulators, and their involvement in the processes of development, differentiation, cell proliferation, cell death, chromosomal segregation and metabolism. The discovery of microRNAs in species ranging from Caenorhabditis elegans (C. elegans) to humans, and their regulatory functions, opened a new tier of understanding of gene expression. In addition, the study of RNA interference (RNAi) and its mechanisms propelled the elucidation of various functions of microRNAs. The development and brisk progress in microRNAs research over the past few years allowed us to comprehend the genomics at a newer scale. Therefore, it is now an ideal time to examine the available evidence in a systematic way in order to know where this impressive field is going.
MicroRNAs: From Basics to Disease Biology is mainly intended for readers in thefields of molecular cell biology, genomics, biotechnology and molecular medicine. This book may be useful in more advanced graduate level courses. This book, which focuses on the concepts of microRNA biology, key implications in various forms of diseases, and applications in diagnostic and drug development, consists of thirty-eight chapters, grouped into six sections. Most of the chapters are written by the original discoverers or their associated scientists from academia, biotech and pharma, exclusively from the microRNomics field.
Although several reviews have been published covering these tiny molecules, there is no single volume currently available in the marketplace. This is the first book that integrates the academic science with industrial applications from the diverse role of microRNAs development to disease biology, covering bioinformatics, quantitation, prognosis and diagnostics as well drug development and validation. This book will serve as a reference for graduate students, post-doctoral researchers, and professors from academic research institutions who wish to initiate microRNA research in their own laboratories. Additionally, this book will serve as a descriptive and in-depth scientific explanatory analysis for executives and scientists in research and development from biotech and pharmaceutical companies. It will provide a valuable executive scientific summary for investors and those responsible for business development in the life sciences, who need to keep abreast with the microRevolution. Most importantly, my hope is that this volume will serve both as a prologue to the field for newcomers, as well as an update to those already active in the field.
Sydney Brenner from the MRC Molecular Biology Laboratory in Cambridge opened the field of C. elegans biology (mid 1970s), when the lin-4 mutant was originally discovered. Later (his post-doctoral fellow) Howard Robert Horvitz from Massachusetts Institute of Technology isolated lin-14. Although both these genes were discovered in the study of developmental timing defects of the worm their nature and functions were not explored until Horovitz’s post doctoral fellows, Ambros and Ruvkun, studied them. Fourteen years ago, Victor Ambros and his colleagues from Dartmouth Medical School identified for the first time that lin-4 was the first short non-coding microRNA that regulates gene expression in C. elegans. At the same time Gary Ruvkun and his colleagues from the Harvard Medical School demonstrated lin-14 to be the first microRNA target gene. Together, these two seminal discoveries identified a novel mechanism of post-transcriptional gene regulation. A successful initial collaboration and subsequent independent contributions by Ambros and Ruvkun paved the way to our current understanding of microRNA biology. The microRNAs world did not take off until the discovery of RNAi, and let-7, a second microRNA discovered by Ruvkun and his colleagues in 2000. The highly conserved nature of let-7 attracted a great deal of attention to microRNAs research and since then many groups have identified microRNAs in various organisms, from protozoans to humans. The paradigm from the heterochromic lin-4/lin-14 genes remains the model; miRNAs have now been shown to control mRNA abundance in plants, and are thought to regulate many more steps beyond translation. Despite considerable attention having been paid to microRNAs over the past five years, a lot of questions remain unanswered. The mechanism of action of microRNAs is still controversial and only a few in vivo functions of microRNAs have been demonstrated in any organism to date. In this book I intend to bring the various aspects of microRNA research together, including the biology, applications, their role in stem cell and disease biology. As the field of microRNomics is rapidly growing, any volume that describes the material might be little outdated. The role of microRNAs in immunology, cardiology, diabetes, and unicellular organisms has been recently reported, after I communicated all the original chapters to the press. Therefore I have added two new chapters reviewing these topics at the end of this book.
As Ambros mentioned in an earlier paper, the lin-4 story is one of persistent curiosity, luck, timing, and the generosity of colleagues. Indeed, it is a key breakthrough in the modern molecular biology. In the coming years, microRNA research will necessitate the rewriting of our textbooks and alter the basic concept of the central dogma. In the near future we will be able to use microRNA-based molecular signatures or panels of multiplexed microRNA biomarkers to identify whether a tumor is malignant or benign, its site of origin, its prognostic subtype, and even predict its response to therapy. Clearly, high-throughput approaches and bioinformatics will have a primary role in the future of clinical oncology and pathology. In addition, microRNAs will be used in targeted cardiovascular therapy and they will be a central tenet in ‘translational medicine’. To fully realize the potential of microRNAs as biomarkers to aid in drug development, industry must implement best practices for biomarker development, and promote translational research strategies. The biggest obstacles to turning discovery from bench to bed-side are not only, in the advancement of technology, but also the necessary regulatory approvals. In a nutshell, microRNA array technology has the potential to accommodate all required assay formats on one testing platform, and to provide better reagents for pathological diagnosis in the future. It is hoped that by fully understanding the functions of microRNAs in various forms of human diseases and their subsequent development as theranostics, so we will be the new era of ‘translational medicine’.
Many people have contributed to making my involvement in this project possible. I thank my teachers throughout my life for their excellent teaching, guidance and mentorship, which caused me to become a scientist. I am thankful to all of the contributors to this book. Without their commitment this book would not have emerged for the reader. Many people have had a hand in the launch of this book. Each chapter has been passed back and forth between the authors for criticism and revision, so that each chapter represents a joint composition. Thanks to you, the reader, who makes the hours I have spent putting together this volume worthwhile, if you find value in the hours you spend with this book. I am indebted to the staff of Cambridge University Press, and in particular Katrina Halliday for her generosity and efficiency throughout the editing of this book; she truly understands the urgency and need of this volume. I would specially like to thank Nobel Laureate Sidney Altman and Victor Ambros for their kindness and support in writing the Forewords to this book. Last, but not least, I thank my two wonderful sons, Raakish and Raghu, for their understanding and cooperation during the development of this book.
A portion of the royalties will be contributed to the Dr Appasani Foundation, a non-profit organization devoted to bring social change through education of youth in developing nations.
I Discovery of microRNAs in various organisms
The microRNAs of C. elegans
Ines Alvarez-Garcia and Eric A. Miska*
Discovery
MicroRNA (miRNA) is a class of short non-coding RNA that regulates gene expression in many eukaryotes. MicroRNA was first discovered in Caenorhabditis elegans by Victor Ambros’ laboratory in 1993 (Lee et al., 1993). At the same time Gary Ruvkun’s laboratory identified the first microRNA target gene (Wightman et al., 1993). Together, these two seminal discoveries identified a novel mechanism of post-transcriptional gene regulation. The history of these events has been reported recently, as remembered by the main researchers involved (Lee et al., 2004; Ruvkun et al., 2004). The realization of the importance of the discovery of microRNA, however, took about seven years and was preceded by the identification of a second microRNA in C. elegans by the Ruvkun and Horvitz laboratories and also by the rise in interest in another class of short RNA, siRNA, involved in the process of RNAi and related phenomena in plants and animals (Hamilton and Baulcombe, 1999; Zamore et al., 2000). Although the discovery of microRNA was unexpected, theoretical work on the mechanism of the lac repressor by Jacob and Monod had postulated a microRNA-like mechanism forty years earlier, see Figure 1.1 (Jacob and Monod, 1961).
Biogenesis and mechanism of action
From studies in C. elegans, Drosophila melanogaster and mammalian cell culture a model for microRNA biogenesis in animals is emerging (see Figure 1.2a and Figure 1.2b): microRNAs are transcribed by RNA polymerase II as long RNA precursors (pri-miRNAs) (Kim, 2005), which are processed in the nucleus by the RNase III enzyme Drosha and DGCR8/Pasha, to form the approximately 70 base pre-miRNA (Lee et al., 2003; Denli et al., 2004; Gregory et al., 2004; Han et al., 2004; Landthaler et al., 2004). Pre-miRNAs are exported from the nucleus by Exportin-5 (Lund et al., 2004) and processed by the RNase III enzyme, Dicer, and incorporated into an Argonaute containing silencing complex (RISC) (Du and Zamore, 2005). From this
Image not available in HTML version |
Figure 1.1 Models for the regulation of protein synthesis. Reproduced from Jacob and Monod (1961). Model II depicts a microRNA-like mechanism. Both models were proposed for the lac repressor and turned out to be false, as the lac repressor is a protein transcription factor. However, model I and II may underlie short RNA mediated gene regulation at the transcriptional and post-transcriptional level respectively.
general model a number of factors have been directly implicated in microRNA function in C. elegans. Drsh-1 (Drosha) and Pash-1 (DGCR8/Pasha) are required for pri-miRNA processing (Denli et al., 2004). Dcr-1 (Dicer) is required for pre-miRNA processing (Grishok et al., 2001; Ketting et al., 2001; Knight and Bass, 2001). Interestingly, two Argonaute family members, Alg-1 and Alg-2 are also required for the generation of the mature microRNA from pre-miRNA, suggesting that at least in C. elegans Argonaute proteins not only play roles in microRNA mechanism of action but also microRNA biogenesis (Grishok et al., 2001). In animals microRNAs are thought to function through the inhibition of effective mRNA translation of target genes through imperfect base-pairing with the 3′-untranslated region (3′UTR) of target mRNAs (see Figure 1.2c) (Bartel, 2004; Pillai et al., 2005). However, alternative mechanisms of action include direct mRNA cleavage (Mansfield et al., 2004; Yekta et al., 2004) and mRNA stability (Bagga et al., 2005; Jing et al., 2005; Giraldez et al., 2006). In vivo microRNA targets are largely unknown, but estimates range from one to hundreds of target genes for a given microRNA, based on microRNA target predictions using a variety of bioinformatics approaches (Lewis et al., 2003; Stark et al., 2003; John et al., 2004; Rajewsky and Socci, 2004; Brennecke et al., 2005; Farh et al., 2005; Lewis et al., 2005; Stark et al., 2005; Xie et al., 2005; Lall et al., 2006).
Image not available in HTML version |
Figure 1.2 MicroRNA biogenesis and mechanism of action. A current model of microRNA biogenesis and mechanism of action. This model is a generic model of the biogenesis of microRNAs in animals. The lin-4 miRNA and the lin-14 mRNA are shown as examples. The corresponding factors that have been implicated in the biogenesis of microRNAs in C. elegans are Drsh-1 (Drosha), Pash-1 (Pasha), Dcr-1 (Dicer) and Alg-1/2 (Ago).
MicroRNA gene identification and expression analysis
The first microRNAs were identified through forward genetic screens in C. elegans, as is described below. However, the majority of microRNAs in C. elegans and other organisms were identified using molecular biology techniques and bioinformatics (Lau et al., 2001; Lee and Ambros, 2001). Strict criteria for the identification and naming of microRNAs have been agreed upon (Ambros et al., 2003a). Importantly, microRNAs need to be derived from stem-loop precursors and verified experimentally by either northern blotting or polymerase chain reaction using specific primers, unless they represent clear orthologs of microRNAs in related species (Lau et al., 2001; Lee and Ambros, 2001; Grad et al., 2003; Lim et al., 2003; Ohler et al., 2004). The public database that has been established for microRNAs, miRBase, currently lists 115 C. elegans microRNAs (release 8.0)(Griffiths-Jones, 2004; Griffiths-Jones et al., 2006). However, of these only 98 microRNAs are likely to be real (Lim et al., 2003; Ohler et al., 2004). Of these, at least 40% are conserved in humans (Lim et al., 2003). In addition, other classes of short RNAs, distinct from microRNAs have been identified in C. elegans, endogenous siRNAs and tiny non-coding RNAs (tncRNAs) (Ambros et al., 2003b; Lee et al., 2006). It is likely that the number of short RNAs identified in C. elegans will continue to grow and it would not be surprising if additional microRNAs were identified.
To aid the study of microRNA function, analysis of microRNA expression may provide useful information. The temporal expression of many microRNAs in C. elegans has been determined previously using northern blotting (see Table 1.1) (Lee et al., 1993; Reinhart et al., 2000; Lau et al., 2001; Lee and Ambros, 2001; Lim et al., 2003; Grad et al., 2003; Ohler et al., 2004). These studies revealed that possibly one third of C. elegans microRNAs are differentially expressed during larval development. However, a large number of microRNAs appear to be expressed throughout embryonic and larval stages and adulthood. It would be interesting to extend these studies to include embryonic stages and a collection of mutant backgrounds. The availability of microRNA microarray technology should aid future microRNA expression studies (Krichevsky et al., 2003; Miska et al., 2004). In addition to information regarding temporal expression of microRNAs, spatial information would be very informative. Spatial expression of microRNAs in C. elegans so far has been restricted to indirect methods such as promoter GFP fusion transgenes (Johnson et al., 2003). It remains an open question if in situ approaches to directly detect microRNAs using oligonucleotide probes that have been successful in plants, flies and fish will also be applicable to C. elegans (Kidner and Martienssen, 2004; Sokol and Ambros, 2005; Wienholds et al., 2005).
MicroRNA target predictions
The first microRNA targets, lin-14, lin-28 and lin-41 mRNAs were identified through genetic interactions with the lin-4 and let-7 microRNAs respectively (Ambros, 1989; Ruvkun and Giusto, 1989). However, a number of microRNA target prediction algorithms have been described (Lewis et al., 2003; Stark et al., 2003; John et al.,
© Cambridge University Press