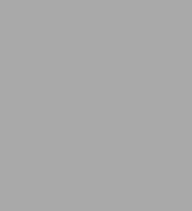
Molds, Molecules, and Metazoa: Growing Points in Evolutionary Biology
194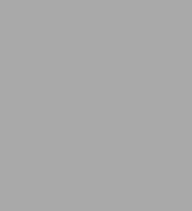
Molds, Molecules, and Metazoa: Growing Points in Evolutionary Biology
194Paperback
-
PICK UP IN STORECheck Availability at Nearby Stores
Available within 2 business hours
Related collections and offers
Overview
The scope of this volume ranges from macroevolutionary patterns in the Precambrian to molecular evolution of the genome. Major themes include the origin and maintenance of variation and the causes of evolutionary change. Chapters on paleontology, ecology, behavior, development, and cell and molecular biology are contributed by Jim Valentine, Graham Bell, Mary Jane West Eberhard, Leo Buss, Marc Kirschner, and Marty Kreitman. The book contains an introductory chapter by John Bonner, whose seminal work is honored here.
Originally published in 1992.
The Princeton Legacy Library uses the latest print-on-demand technology to again make available previously out-of-print books from the distinguished backlist of Princeton University Press. These editions preserve the original texts of these important books while presenting them in durable paperback and hardcover editions. The goal of the Princeton Legacy Library is to vastly increase access to the rich scholarly heritage found in the thousands of books published by Princeton University Press since its founding in 1905.
Product Details
ISBN-13: | 9780691602813 |
---|---|
Publisher: | Princeton University Press |
Publication date: | 07/14/2014 |
Series: | Princeton Legacy Library , #193 |
Pages: | 194 |
Product dimensions: | 6.00(w) x 9.10(h) x 0.50(d) |
Read an Excerpt
Molds, Molecules, and Metazoa
Growing Points in Evolutionary Biology
By Peter R. Grant, Henry S. Horn
PRINCETON UNIVERSITY PRESS
Copyright © 1992 Princeton University PressAll rights reserved.
ISBN: 978-0-691-08768-9
CHAPTER 1
Evolution and the Rest of Biology: The Past
JOHN TYLER BONNER
My passion for biology spans over fifty years, and during the course of those fifty-odd years two things have happened: one to me and one to the world. In my own case, as the years progressed, I have become increasingly obsessed with the idea that evolution by natural selection is the most useful, the most important, the most all-enveloping concept in all of biology. That has been my own evolution. In the case of the world at large, there have been the most staggering advances and rapid changes of monumental importance in biology during this period. Here I would like to trace, with a very light brush, what those changes have been. My purpose is to bring the reader rapidly across the terrain of this half century, right to the edge of the cliff, to the beginning of the unknown void of the next fifty years. I do this keeping in mind that we are not just talking about the advances in any particular field, but how those advances are held together by the glue of evolution. The chapters in this volume show what some gifted young scholars, preeminent in their respective fields, can see as they peer over the precipice and look into the future.
Paleontology
In his On the Origin of Species, Darwin argued that one of the reasons it is difficult to trace the lineage of species through the fossil record is that the record is so imperfect—there are so many gaps. What he said then could be justifiably said today, but the difference in our knowledge is enormous. Today, the total number of fossils known is vast compared to what was available in the middle of the last century. Furthermore, with our methods of dating fossils by combining the geological knowledge of the sequence of strata with methods using radioisotopes, we have in recent years developed a remarkably accurate time course for our fossil sequence. Therefore, we have done much to fill in the gaps deplored by Darwin but, like the pursuit of fractals, we find that with increased magnification and precision the gaps may be smaller, but they are still there, and, if anything, they are more numerous than before.
There have been some very exciting discoveries in the never-ending search for new fossils. For instance, there was the remarkable finding of fossils of very early, primordial bacteria. There was also the discovery (and, more recently, the interpretation) of the ancient fossils of the Burgess Shale which reveal the soft parts—not just the skeletal armor—of a wide and fascinating variety of forms of invertebrates. These discoveries, and others like them, have provided for a steady accumulation of new information, new facts about the plants and animals of the past.
Significant as these discoveries are, the great advances came from making useful generalizations from these facts; in a sense, they are the grist for the all-important mill of insightful models. Certainly, even in the last century, there have been attempts to make broad generalizations about trends in fossil sequences, in fossil history. There was Cope's rule of trends in size increase and other such generalizations which are still of use to us today. The revolution, however, came from the work of the paleontologist G. G. Simpson. He was the first to look into the matter of rates of evolution. He was able to calculate the rates of origin of new species, new genera, new orders, and so forth, at different periods of earth history, as well as rates of extinction, and in this way obtain a picture of what he called in the titles of one of his influential books "the major features of evolution" and, in another, "tempo and mode in evolution." He could use these quantitative methods for the study of rates of change because the accumulation of facts about fossils had reached a sufficient level of detail, and with these facts he was able to weave together some generalizations of major significance.
It is this approach that has dominated paleontology in recent years. Sometimes it has produced generalizations that are in complete harmony with population genetics and neo-Darwinism. This certainly was the view of G. G. Simpson. Recently there has been a heated debate as to whether evolutionary change is gradual or involves great bursts followed by periods of stasis (the so-called punctuated equilibria model). Sometimes there has been more heat than light generated in this debate and, as is so often the case, both sides are no doubt correct. In any event, there is nothing to seriously shake the foundation of evolution by natural selection, although there could be special features of the speed of change in speciation that we do not yet fully understand. This is the edge of the precipice of interpreting the fossil record: we have new tools and new methods. The future should be exhilarating.
Ecology
In 1941, just after I received my B.S. degree, I spent a good part of one summer in Barro Colorado Island. It was a great awakening for me. In the first place, callow as I was, I was absolutely overwhelmed by the richness of the tropical rain forest. It was far beyond anything my imagination or reading had ever conjured up in my mind. The other was a week's visit there by Professor Victor Shelford, then an old man (or at least it seemed so to me at the time) who was well known for his ecology textbook. We had many conversations about biology and ecology in which I gained a clear impression that in his view one must not tamper with nature and experiments were bound to be misleading because they were not "natural." What the ecologist should do is try to appreciate the great complexity of the biosphere and record it as accurately and as systematically as possible. Since I was a confirmed experimentalist, I spent some time vigorously arguing with him; but despite our many disagreements (which included politics and Franklin Delano Roosevelt), I did see his point about complexity and the extraordinary interrelations and interdependence among all the parts of an ecosystem.
The great change came in the 1950s and 1960s, when G. E. Hutchinson and, more especially, his student Robert MacArthur began to apply mathematical models in an attempt to make simplified generalizations about the great complexity of ecological systems. As we all know, MacArthur was remarkably successful in this enterprise and did indeed spawn a revolution. Testimony to its success was the great cry of resentment from the traditional ecologists of the older school, who felt that the important aspect of an ecosystem was its complexity; anything that tried to simplify it was working in the wrong direction. But the MacArthurian insights were powerful and they carried the day. Furthermore, they greatly helped to link ecology with evolution, which he and G. E. Hutchinson and others did so much to encourage.
Since that golden age there has been a refinement of the models. Many of the original ones have even been supplanted, as MacArthur predicted they would be. It has also been a period of exponential proliferation of models; the approach has been so fruitful that it has seeped into every corner of ecology and across the boundary into behavior and evolution. There is no doubt that this has been one of the most successful cases so far in biology, where mathematics has helped to give insight into complex mechanisms and interactions of many kinds. We can easily imagine that the same will be true for other fields of biology, but for ecology it has been a fertile period for the use of mathematics as a way to shed light on a difficult ecological problem. The days of "thick description" are happily gone. This is where we are now, at the edge of the precipice that leads to the future. I cannot imagine that the next steps will be more of the same; perhaps we are ready for a new insight or a new approach.
Behavior
There always seemed to be some confusion in Darwin's mind about what he called instinct. It no doubt stemmed from his inability to account for the mechanism of inheritance. In a number of passages in his writings he makes it clear that he sees a difference in how instincts might be inherited, in contrast to morphological changes, but he is never explicit on what these changes might be. Yet he frequently seems to imply that instincts are inherited in a rather Lamarckian fashion.
Well into this century the problem occupied the minds of many and led to a great dichotomy: those who became enamored with the idea that behaviors (instincts) were inherited, of which the eugenicists were the most notorious in that they were considered synonymous with bigots, and those who said that the environment and learning were the sole determinants of behavior. This became the rather sad and pointless nature-nurture debate. When I took elementary biology, the Jukes and the Kalikaks, families that spawned an exceptionally large number of criminals over a number of generations, were proof that delinquency was indeed inherited. Hardly ten years later this "fact" was expunged from all biology textbooks, only to reappear in sociology texts as evidence that the environment had a profound effect on behavior. In fact, the word "instinct" itself became disreputable, because how could one ever know the genetic component in any behavioral act?
The revolution came with the work of Konrad Lorenz and Niko Tinbergen and others in the birth of ethology. They made it possible, by the carefully controlled study of animals in nature, to be able to distinguish between instinctive and learned behaviors, and "instinct" was able to regain its former respectable reputation. In this etiological revival there was an immediate concern with how instinctive behaviors were controlled by natural selection, and a convincing case was made to show that the behaviors, which were automatic and clearly inherited, did endow fitness, and therefore reproductive success, to those individuals that inherited the behaviors.
The culmination of this approach came in the enormously important inspiration of W. D. Hamilton in the 1960s—that the key to understanding the evolution of social behavior was the genetic relatedness of the individuals involved. Instinctively helping kin could appear to be altruistic, but in fact there was also a selfish component to the behavior, so that by such apparent altruism one made it more likely that the genes one shared with close relatives would be passed on to the next generation. This raised a great interest in animal societies, which came to a peak in the publication by E. O. Wilson in 1975 of his important book, Sociobiology. Unfortunately it was met not only with acclaim by biologists in general, but with vitriolic abuse from Marxists who used it to revive the moribund nature-nurture problem and all its sinister possibilities for eugenics. This attack had nothing to do with the main body of the work, but only considered the implications for human sociobiology, which was not part of Wilson's central agenda, nor is it to this day a subject tractable for sensible study. Perhaps the only good that has come out of the human aspects of sociobiology is to stimulate the beginning of a greater awareness of the interactions between cultural and genetic inheritance, a field that is still in its infancy.
Much of the recent study of interactions in animal societies has, quite obviously, centered around means of communication among individuals. Some of the most significant studies stem from the social insects, for they have so many different kinds of social groupings, and some of them are so elaborate. For example, Karl von Frisch showed that scout bees could tell the other foraging worker bees both the direction and the distance of a source of nectar, a discovery so surprising that few believed the story when they first heard the rudiments of it. And it was also the hymenoptera that gave W. D. Hamilton the idea that has come to be known as kin selection.
The fact that behavior and evolution are intimately entwined has never been more evident than it is now. For many reasons—most of them made possible only in the last fifty years—we see the problem far more clearly than Darwin could. This is where we stand on the cliff looking out into the future.
Development
In the case of development, there are two very distinct traditions. One is the explanation of how plants and animals develop: this is the "mechanics of development" of Wilhelm Roux in the latter part of the last century and the "casual embryology" of Albert Dalcq in the early part of this century. The other is the relation of development to evolution, a tradition that goes back at least to Karl Ernst von Baer in the early 1800s, and later, as the nineteenth century progressed, many others came to make important contributions to the subject. They include Charles Darwin, August Weismann, and Ernst Haeckel, and early in the twentieth century there were Walter Garstang and Gavin de Beer. (The roots go even farther back, as Stephen J. Gould shows admirably in his book, Ontogeny and Phytogeny.) Let me discuss briefly both approaches—the how and the why of development—and then show how they seem to have come closer together in recent years.
Twenty or thirty years ago it was often said that the golden age of developmental biology peaked early in the twentieth century, with the discovery by Hans Spemann of the "organizer" in the amphibian embryo, which demonstrated that one part of an embryo could send chemical messages to another and in this way call forth or induce new structures in undifferentiated tissues. It was the beginning of a great search for what are now called "morphogens," chemical signals which coordinate and orchestrate the development of an organism. In plants, the discovery of auxin and other growth hormones has been of major importance, and although the chemical identification of the morphogens in animal embryos has been slow, recently we have seen major new discoveries, such as the role of retinoic acid in limb development in vertebrates. The other modern feature of this offshoot from Spemann's work has been the use of mathematical modeling. It was given some initial sparks in the work of Nicolas Rashevsky, but generally the broad concepts of reaction-diffusion mathematics and how they might apply to development are attributed to Alan Turing in his famous paper of 1952. From all this we can see that while Spemann's work opened up a wide avenue of research, that avenue has been productive more or less continuously for many years.
The more recent development, and one of at least equal, and probably greater, importance, is the rise of developmental genetics. It has been known for a very long time that much of the information for development is stored in the genes of a fertilized egg, but what has been unclear until recently is how the genes impart their information to the embryo. The origins of this approach come partly from classical genetics and partly from the great rise of molecular genetics following the mega-discovery by James Watson and Francis Crick of the structure of DNA, which led to our understanding of how the genetic code works and how the genes designate the synthesis of specific proteins.
With these tools and associated clever experimental methods, it is now possible to identify the structure of specific genes, the structure of the proteins they produce, where the proteins are located in the embryo, and even where they are located in the cells of the embryo. With this incredibly powerful armory of techniques it is now possible to trace in great detail the chemical course of events in the development of an organism. This work is being done with notable success in the development of the fruit fly Drosophila and the nematode Caenorhabditis, which are being intensively studied in many laboratories. They make a particularly happy choice because the early development of Drosophila is to a considerable extent regulative, and the gene products are in many cases themselves morphogens that signal from one region of the embryo to another. In contrast, the development of Caenorhabditis is relatively mosaic and the cell lineages are fixed. Yet in both there are instances where individual cells can signal to their immediate neighbors, and in some cases we know the structure of genes and the structure of the signal proteins as well as that of the receptor protein. The future of "how" development takes place could not look brighter than it does at the moment.
(Continues...)
Excerpted from Molds, Molecules, and Metazoa by Peter R. Grant, Henry S. Horn. Copyright © 1992 Princeton University Press. Excerpted by permission of PRINCETON UNIVERSITY PRESS.
All rights reserved. No part of this excerpt may be reproduced or reprinted without permission in writing from the publisher.
Excerpts are provided by Dial-A-Book Inc. solely for the personal use of visitors to this web site.
Table of Contents
List of ContributorsPreface
1 Evolution and the Rest of Biology
2 Lessons from the History of Life
3 Five Properties of Environments
4 Behavior and Evolution
5 The Middle Ground of Biology: Themes in the Evolution of Development
6 Evolution of the Cell
7 Will Molecular Biology Solve Evolution?
8 Overview: Variation and Change
References
Index