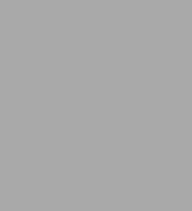
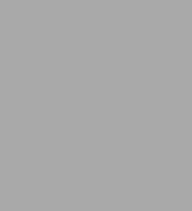
eBook
Available on Compatible NOOK devices, the free NOOK App and in My Digital Library.
Related collections and offers
Overview
Product Details
ISBN-13: | 9781935622307 |
---|---|
Publisher: | Scott & Nix, Inc. |
Publication date: | 11/17/2011 |
Sold by: | INDEPENDENT PUB GROUP - EPUB - EBKS |
Format: | eBook |
Pages: | 77 |
File size: | 2 MB |
About the Author
Read an Excerpt
More Brain Cuttings
Further Explorations of the Mind
By Carl Zimmer
Scott & Nix, Inc.
Copyright © 2011 Carl ZimmerAll rights reserved.
ISBN: 978-1-935622-31-4
CHAPTER 1
A Body Fit for a Gigantic Brain
In 1758 the Swedish taxonomist Carolus Linnaeus dubbed our species Homo sapiens, Latin for "wise man." It's a matter of open debate whether we actually live up to that moniker. If Linnaeus had wanted to stand on more solid ground, he could have instead called us Homo megalencephalus: "man with a giant brain."
There's no disputing that the human brain is freakishly big. The average human brain weighs in at about three pounds, or 1,350 grams. Our closest living relatives, the chimpanzees, have less than one-third as much brain — just 384 grams. And if you compare the relative size of brains to bodies, our brains are even more impressive.
As a general rule, mammal species with big bodies tend to have big brains. If you know the weight of a mammal's body, you can make a fairly good guess about how large its brain will be. As far as scientists can tell, this rule derives from the fact that the more body there is, the more neurons needed to control it. But this body-to-brain rule isn't perfect. Some species deviate a little from it. A few deviate a lot. We humans are particularly spectacular rule breakers. If we were an ordinary mammal species, our brains would be about one-sixth their actual size.
Scientists have put forward different explanations for why we ended up with a big brain. One idea, championed by University of Oxford psychologist Robin Dunbar, is that when social life gets complicated, natural selection favors a bigger brain. In a primate species with large societies, an individual may need to keep track of dozens of other individuals who might be allies or enemies. A primate that can manage a big social network well has better odds of surviving and reproducing. But keeping tabs on one's social life is mentally demanding.
Dunbar and his colleagues have measured the cost of social thinking in a series of experiments. In one test, they found that people take longer to answer questions that require them to think about what's going on in other people's minds. The more mind-reading a question requires, the more it activates the brain. Evolving a bigger brain may have given our ancestors more neurological horsepower to handle these tasks.
Daniel Sol of the Center for Terrestrial Ecology and Applied Forestries in Barcelona has put forward a different idea: we evolved big brains because they helped our ancestors solve problems in new environments. Sol tested this idea by looking at animals that have been introduced by humans into new habitats. Some immigrants have successfully settled in their new homes, while others have simply become extinct. Comparing successful and unsuccessful invaders, he found that in both birds and mammals, big-brained species are more likely to be successful than are small-brained ones. This research suggests that larger brains are more adept at problem solving, which translates into a better chance of survival.
It is possible that both Dunbar and Sol are at least partially correct. But no matter how the debate resolves, a puzzling question remains: If big brains are so useful, then why are they relatively rare? The answer is that nothing in nature comes for free — and where the brain is concerned, the cost can be enormous. In fact, scientists are discovering that the human body has dramatically reorganized itself to cope with the burden of an oversize brain.
In 1995 Leslie Aiello, then of University College London, and Peter Wheeler of Liverpool John Moores University offered the first possible reason for the rarity of big brains. Neurons, they pointed out, have a voracious appetite. They require lots of energy to produce their voltage spikes and to release neurotransmitters. They get that energy from oxygen and food, mostly glucose. A three-pound human brain burns up to 20 times as many calories as three pounds of muscle.
We cannot ignore this demand, even for a moment. A few minutes without oxygen may not do too much damage to our muscles but can irreparably harm the brain. The brain also requires a constant supply of food. Twenty-five percent of all the calories you eat each day end up fueling the brain. For a newborn infant, with its little body and relatively large and fast-growing brain, that figure leaps to 87 percent.
The brains of our ancestors more than 6 million years ago did not impose such brutal demands. Our forebears stood only about as tall as a chimpanzee and had brains the size of a chimp's. For the next 4 million years, our ancestors remained small-brained. Then, around 1.8 million years ago, Homo erectus evolved. The first members of our genus that looked like us, H. erectus stood about as tall as modern humans, with brains that weighed around 900 grams. A half-million years ago, the brains of our ancestors started to grow again; 200,000 years ago they finally reached about the same weight as Homo sapiens brains today.
Aiello and Wheeler noted that this dramatic increase in brain size would seem to have required a dramatic increase in metabolism — the same way that adding an air-conditioning system to a house would increase the electricity bill. Yet humans burn the same number of calories, scaled to size, as other primates. Somehow, Aiello and Wheeler argued, our ancestors found a way to balance their energy budget. As they expanded their brains, perhaps they slimmed down other organs.
The scientists compared the sizes of organs in humans and other primates. Relatively speaking, our liver is about the same size as a baboon's. Our heart is on par with a gorilla's. But our guts have shriveled. They weigh only 60 percent of what you'd expect in a primate of our size. Intestinal cells also need a lot of energy, because they are highly innervated. Losing such a big portion of their guts could have allowed our ancestors to compensate for much of the brain's extra energy demand.
Aiello and Wheeler christened their idea "the expensive tissue hypothesis." To test it, they compared the size of brains and guts in a range of primate species. They found that the bigger a primate's brain relative to the species's overall body size, the smaller the guts tend to be. This consistent trade-off suggested that trimming our guts was essential to supersizing our brains.
Then William Leonard, a biological anthropologist at Northwestern University, put the expensive tissue hypothesis to a new test. Instead of correlating brain and gut size across primate species, Leonard decided to look at mammal species overall. Beyond the primates, he found, there existed no correlation whatsoever between brain size and gut size.
This suggested that the gut-shrinking phenomenon within the primate groups was probably too subtle to explain our increase in brain size completely. Something else had to be going on as well. That something, Leonard says, is diet. After studying the diets of primate species and tallying the quantity and quality of food consumed, Leonard found a switch from lower-energy diets of bark and leaves to higher-energy cuisines of seeds, tubers, and meat in the brainier species. As brain-to-body ratio increases, presumably, the denser calories supply the additional needed fuel.
Greg Wray, an evolutionary biologist at Duke University, is finding secrets to big brains in an entirely different place: the human genome. One of the genes involved in feeding the big brain, called SLC2A1, builds a protein for transporting glucose from blood vessels into cells. It is vital to the brain's well-being. Mutations that reduce the number of transporter proteins in the brain lead to disorders such as epilepsy and learning disabilities. If one copy of the SLC2A1 gene is completely dysfunctional, the results are devastating: The brain develops to only a portion of its normal size. If neither copy of the gene works, a fetus simply dies.
Wray and his colleagues compared SLC2A1 in humans and other animals. They discovered that our ancestors acquired an unusually high number of mutations in the gene. The best explanation for that accumulation of mutations is that SLC2A1 experienced natural selection in our own lineage, and the new mutations boosted our reproductive success. Intriguingly, the Duke team discovered that the mutations didn't alter the shape of the glucose transporters. Rather, they changed stretches of DNA that toggled the SLC2A1 gene on and off.
Wray guessed that these mutations changed the total number of glucose transporters built in the human brain. To test his theory, he looked at slices of human brain tissue. In order to make glucose transporters, the cells must first make copies of the SLC2A1 gene to serve as a template. Wray discovered that in human brains there were 2.5 to 3 times as many copies of SLC2A1 as there were in chimpanzee brains, suggesting the presence of more glucose transporters as well.
Then he looked at glucose transporters that deliver the sugar to muscles. The gene for these muscle transporters, called SLC2A4, also underwent natural selection in humans, but in the opposite direction. Our muscles contain fewer glucose transporters than in chimps' muscles. Wray's results support the notion that our ancestors evolved extra molecular pumps to funnel sugar into the brain, while starving muscles by giving them fewer transporters.
Becoming Homo megalencephalus was hardly a simple process. It was not enough for evolution to shrink our gut and shift our diet. It had to do some genetic engineering, too.
CHAPTER 2The Genius of Athletes
What sets athletes apart from the rest of us? The first answer that jumps to mind is that they look different — think of the fireplug physique of a weightlifter, or the stingray shape of an Olympic swimmer. But athletes have remarkable qualities aside from muscles mass and lung capacity. Their brains are different, too. Athletes are masters of decision-making. They make good decisions about how to move their bodies, and they make those decisions fast. A basketball player sees an oncoming opponent raise the ball over her head and has to make a choice: jump to block the shot, or stay on the ground so as not to be fooled by a pump fake. Along with conscious decisions like this one, athletes make many others without a trace of awareness. Each second, an athlete's brain must decide which commands to send out to the body — which muscles to contract, and which to relax.
Athlete have to make decisions quickly, but they can't just respond automatically with a simple reflex. There isn't enough room in the human brain to store every automatic response to every possible situation an athlete might encounter. Even a sport as seemingly straightforward as pistol-shooting is surprisingly complex. Pistol-shooters don't have to play in sandtraps like golfers, or battle an opponent like fencers. They just point and shoot. Yet, in that simple act, a shooter must make many decisions in a split-second, such as what angle to hold the elbow and how tight to contract the shoulder muscles. When a pistol shooter fires a series of shots, all those variables turn out differently from one shot to the next.
In 2008, two neuroscientists developed a model to explain how the brain decides how to move the body. Reza Shadmehr of Johns Hopkins University and John Krakauer of Columbia University proposed that the brain carries out a sophisticated kind of information processing they call optimal feedback control. The brain begins by setting a goal — pick up the fork, say, or deliver the tennis serve — and calculates the best course of action to reach it. As the brain starts issuing commands, it also begins to make predictions about what sort of sensations should come back from the body if it reaches the goal successfully. If there is a mismatch between what it predicts and what it senses, the brain can revise its plan to reduce the error. In other words, the brain does not just issue rigid commands. It merges with the body into a feedback loop. We all use optimal feedback control, but athletes may just be better at it than the rest of us.
Genes may provide some people with better odds of developing an athletic brain. But even the best-endowed prodigies need practice, and a lot of it, to reach that full potential. Practicing a sport transforms the brain, from the very first session. Scientists at the University of Regensberg in Germany were able to document this kind of transformation in a study they carried out on juggling. They had people practice juggling for a week and then took scans of their brains.
Even as practice changes the brain's anatomy, it also helps different regions of the brain talk to one another. Some neurons strengthen their connections to other neurons and weaken their connections to still others. And these changing connections alter the overall pattern of brain activity that occurs when people play sports. When people are beginning to learn a sport, neurons in the front of the brain (the prefrontal cortex) are active. That region is vital for top-down control, which enables us to focus on a task and consider a range of responses. With practice, the prefrontal cortex grows quiet. Our predictions get faster and more accurate, so we don't need so much careful oversight about how to respond.
Several years ago Matthew Smith and Craig Chamberlain of the University of Northern Colorado examined the connection between the quieting of the cortex and athletic ability. They had expert and unskilled soccer players dribble a ball through a slalom course of cones. At the same time, the players were asked to keep an eye on a projector screen on the wall to see when a particular shape appeared. Even with the second task, the seasoned soccer players could dribble at nearly full speed. Unskilled players did much worse than when they were undistracted, however. The disparity suggests that dribbling didn't tax the expert player's prefrontal cortex as heavily, leaving it free to deal with other challenges.
As the brains of athletes become more effecient, they learn how to make sense of a new situation sooner. In cricket, for instance, a bowler can hurl a ball at 100 miles an hour, giving batsmen half a second to figure out its path. In 2006 Sean Muller, then at the University of Queensland in Australia, and his colleagues ran an experiment to see how well cricket batsmen can anticipate a bowler's pitch. For their subjects they chose three types of cricket players, ranging in skill from national champions down to university players. The cricketers watched videos of bowlers throwing balls. After each video was over, they had to predict what kind of pitch was coming and where it would land. In some cases the video was cut off at the point at which the bowler released the ball. In other cases the players got to see only the first step, or the first two steps, that the bowler took while the ball was still in his hand.
Elite cricket players did a much better job than less skilled ones at anticipating the outcome of a pitch. They could make fairly good predictions after watching the bowlers take just a single step, and if they got to see the pitch up to the moment of release, their accuracy improved dramatically. The less skilled players fared much worse. Their early guesses were no better than chance, and their predictions improved only if they were able to watch the pitch until the ball had left the bowler's hand and was in flight.
Predicting the outcome of a task seems to involve the same brain areas that the athlete develops in practice, which would explain why athletes tend to fare better on challenges like these. In a related study, Salvatore Aglioti of Sapienza University assembled a group of people, some of whom were professional basketball players, and scanned their brains as they watched movies of other players taking free throws. Some of the movies stopped before the ball left the player's hands; others stopped just after the ball's release. The subjects then had to predict whether it went through the hoop or not. The pros in the group showed a lot of activity in those regions of the brain that control hand and arm muscles, but in the nonathletes those regions were relatively quiet. It seems that the basketball players were mentally reenacting the free throws in their minds, using their expertise to guess how the players in the movies would perform.
If this emerging view of the athletic brain is correct, then it should be possible to manipulate people's brains and improve their athletic performance. Krakauer and Pablo Celnik of Johns Hopkins decided to run an experiment in which they trained people to do a simple motor task, and alter their brains to help them learn faster. The scientists had volunteers move a cursor horizontally across a screen by pinching a device called a force transducer between thumb and index finger. The harder each subject squeezed, the faster the cursor moved. Each player was asked to move the cursor back and forth between a series of targets, trying to travel the course as quickly as possible without overshooting. The group trained 45 minutes a day for five days. By the end of training, the players were making far fewer errors.
(Continues...)
Excerpted from More Brain Cuttings by Carl Zimmer. Copyright © 2011 Carl Zimmer. Excerpted by permission of Scott & Nix, Inc..
All rights reserved. No part of this excerpt may be reproduced or reprinted without permission in writing from the publisher.
Excerpts are provided by Dial-A-Book Inc. solely for the personal use of visitors to this web site.
Table of Contents
Contents
About the Author,Preface,
A Body Fit for a Gigantic Brain,
The Genius of Athletes,
Switching on the Happy Rat,
How the Brain Wires Itself,
Past Is Prologue,
Decoding the Smile,
A Yardstick for the Nose,
Love Songs and Cockatoo Dances,
The Silly Putty Brain,
Ringing in the Brain,
Where Pain Lives,
The Trouble with Teens,
A Hundred Trillion Connections,
Consciousness, Bit by Bit,
Selected References,