Multiple Drug Resistance in Cancer 2: Molecular, Cellular and Clinical Aspects / Edition 1 available in Hardcover
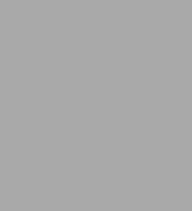
Multiple Drug Resistance in Cancer 2: Molecular, Cellular and Clinical Aspects / Edition 1
- ISBN-10:
- 0792352726
- ISBN-13:
- 9780792352723
- Pub. Date:
- 01/31/1999
- Publisher:
- Springer Netherlands
- ISBN-10:
- 0792352726
- ISBN-13:
- 9780792352723
- Pub. Date:
- 01/31/1999
- Publisher:
- Springer Netherlands
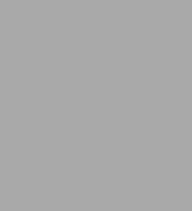
Multiple Drug Resistance in Cancer 2: Molecular, Cellular and Clinical Aspects / Edition 1
Hardcover
Buy New
$219.99-
PICK UP IN STORE
Your local store may have stock of this item.
Available within 2 business hours
Overview
Product Details
ISBN-13: | 9780792352723 |
---|---|
Publisher: | Springer Netherlands |
Publication date: | 01/31/1999 |
Edition description: | Reprinted from CYTECHNOLOGY, 27:1-3, 1998 |
Pages: | 344 |
Product dimensions: | 7.01(w) x 10.00(h) x 0.03(d) |
About the Author
Read an Excerpt
Models for the Mechanism of Drug Transport by P-Glycoprotein
One of the most interesting and controversial issues surrounding P-glycoprotein concerns the mechanism whereby a single transporter molecule can reduce the intracellular concentration of a large number of structurally heterogeneous substrates. Two main hypotheses for the mechanism of drug transport by P-glycoprotein have been suggested, one in which P-glycoprotein interacts with substrates directly to mediate their transport, and one in which an indirect mechanism is responsible. The latter hypothesis, postulated to explain the broad substrate specificity of P-glycoprotein, proposes that P-glycoprotein reduces drug concentration indirectly by altering the cell's electrical membrane potential or by elevating intracellular pH (reviewed in Wadkins and Roepe, 1997). These changes in turn would alter drug partitioning. For example, most of the drug substrates are weakly basic and positively charged at neutral pH, and an increase in intracellular pH would reduce net charge and thus reduce intracellular retention of such compounds. In support of this model, alterations in ion transport characteristics, cytosolic pH and electrical plasma membrane potential have been observed in some cell lines that express P-glycoprotein (reviewed in Roepe, 1995). However, these changes are not found in all MDR1-expressing cells (reviewed in Gottesman and Pastan, 1993). An alternate indirect model postulates that P-glycoprotein acts as an outwardly directed ATP channel, transporting drug molecules out of the cell via an ATP electrochemical gradient (Abraham et al., 1993).
Although indirect mechanisms may contribute to reduced drug accumulation in some cases, such mechanisms are unlikely to explain the high level of resistance observed in MDR cell lines, and most of the experimental data support a direct mechanism of drug transport by P-glycoprotein. Firstly, P-glycoprotein has been shown to directly bind drug analogs; specific regions of the transporter involved in substrate binding have been identified; and mutation of residues in these regions affects substrate binding and substrate specificity (also reviewed in Gottesman et al., 1995). Secondly, as summarized above, highly purified preparations of P-glycoprotein upon reconstitution are able to transport drugs and other substrates, with characteristics reflecting active transport against a concentration gradient. Thirdly, active transport across the membrane of vinblastine and colchicine has been demonstrated by functional expression of mouse Pglycoproteins in yeast secretory vesicles (Ruetz and Gros, 1994a). Substrate transport in the vesicle system was found to occur independently of a proton gradient and was not affected by changes in membrane potential (Ruetz and Gros, 1994a).
While these and other results support a model for the direct transport of substrates by P-glycoprotein, the actual mechanism is not well understood. However, several theories have been forwarded and some significant experimental and conceptual advances made. Because kinetic data implied that P-glycoprotein may be responsible for both increased efflux as well as decreased influx of substrate, the notion arose that P-glycoprotein intercepts its substrates and expels them directly from the membrane before they enter the cytosol. Two of the most intriguing models to account for this property are that P-glycoprotein acts as a `hydrophobic vacuum cleaner' (Gottesman and Pastan, 1993) or as a `flippase' (Higgins and Gottesman, 1992). The vacuum cleaner model was proposed following an elegant study by Raviv et al. (1990). These authors used the photolabile membrane probe 5-[1251]iodonaphthalene-l-azide to show that a direct and specific interaction occurs between Pglycoprotein and its drug substrates in MDR cell membranes. Consistent with this hypothesis, a study with hydrophobic acetoxymethylester derivatives of fluorescent calcium and pH indicators also provided evidence that these transport substrates of P-glycoprotein are removed directly from the plasma membrane (Homolya et al., 1993). The flippase mechanism also argues that substrates gain access to P-glycoprotein directly from the lipid phase. In this model, the role of P-glycoprotein is to transport or `flip' substrate molecules from the inner to the outer leaflet of the membrane bilayer, thus encouraging their export from the cell (Higgins and Gottesman, 1992). However, evidence has also been presented against the hypothesis that drug extrusion occurs from the plasma membrane. Altenberg et al. (1994) found that the unidirectional influx of the P-glycoprotein substrate rhodamine 123 was the same in drug-sensitive and MDR cell lines, suggesting that P-glycoprotein does not intercept the dye but transports it out from the cytosolic compartment.
Both the vacuum cleaner and flippase models envision P-glycoprotein having a pocket or chamber, sufficiently flexible to accommodate substrate structural heterogeneity, in which the molecules are trapped prior to their expulsion. A recent study has provided the first insight into the three-dimensional structure of P-glycoprotein, which appears quite compatible with this suggestion (Rosenberg et al., 1997). The structure was determined to 2.5 urn resolution by electron microscopy and by single particle image analysis. The overall shape is one of a cylinder with a diameter of about 10 nm and height of about 8 nm, appearing toroidal when viewed from the extracellular surface. A large pore is present, open at the extracellular surface with a diameter of about 5 urn but closed at the cytoplasmic face of the membrane with a smaller diameter of 2.5 urn, thus forming a conical chamber. The two transmembrane domains of P-glycoprotein most likely make up the protein surrounding this pore. Other essential features of this structure include a second pore which is asymmetric and opens within the plane of the membrane bilayer. The authors speculate that this may represent the route of entry for substrates from the lipid phase. Two 3 nm lobes at the cytoplasmic face, of a size and orientation appropriate for the two nucleotide binding folds, complete the picture.
Another significant advance in our understanding of the mechanism of action of P-glycoprotein has been made with regards to the catalytic cycle and the stoichiometry of substrate transport and ATP hydrolysis. A scheme has been proposed where ATP hydrolysis occurs in a cyclic fashion, alternating between the two ATP binding sites in each half of P-glycoprotein (Senior et al., 1995). In this scheme, ATP binding to site 1 allows ATP to be hydrolyzed at site 2. This produces a conformational change, resulting in substrate transport and dissociation of the ADP product from site 2. A new molecule of ATP is postulated to then bind to site 2, causing the ATP molecule on site 1 to be hydrolyzed in turn, and the cycle is repeated, with the roles of site 1 and site 2 reversed. This mechanism predicts that one molecule of substrate is transported per molecule of ATP hydrolyzed.
In order to obtain data in support of the models proposed, efforts have been made to determine the stoichiometric relationship between drug transport and ATP hydrolysis. In the reconstitution system of Shapiro and Ling (1995), a value of 50 molecules of ATP per molecule of Hoechst 33342 transported was estimated. However, the authors conceded and emphasized that this low stoichiometry may be due to futile recycling and rebinding of the substrate, together with a high basal ATPase activity. In another study, proteoliposomes containing partially purified Pglycoprotein were used to estimate the quantitative relationship between transport and ATP hydrolysis (Eytan et al., 1996). The rate of valinomycin-dependent A6Rb+ uptake and ATPase activity were measured under identical conditions, and a value of 0.5-0.8 ionophore molecules transported/ATP hydrolyzed was determined. A more recent study also showed a near stoichiometric relationship between drug transport and ATP molecules hydrolyzed (Ambudkar et al., 1997). In this case, the authors were able to compute the maximal rates of vinblastine transport and of vinblastinestimulated ATPase activity. It was calculated that 2.8 molecules of ATP are hydrolyzed per molecule of vinblastine transported, consistent with the metabolic capacity of the cell, and suggesting that drug transport is indeed coupled to ATP hydrolysis.
Table of Contents
P-glycoprotein structure and evolutionary homologies.- Molecular analysis of the multidrug transporter, P-glycoprotein.- Mutagenesis of the putative nucleotide-binding domains of the multidrug resistance associated protein (MRP). Analysis of the effect of these mutations on MRP mediated drug resistance and transport.- Membrane topology of the human multidrug resistance-associated protein (MRP) and its homologs.- A new aspect on glutathione-associated biological function of MRP/GS-X pump and its gene expression.- Intracellular distribution of anthracyclines in drug resistant cells.- Use of ribozymes and antisense oligodeoxynucleotides to investigate mechanisms of drug resistance.- Vault-related resistance to anticancer drugs determined by the expression of the major vault protein LRP.- Topoisomerase I inhibitors and drug resistance.- Mechanisms of resistance to alkylating agents.- Drug resistance and DNA repair in leukaemia.- Nucleotide excision repair and anti-cancer chemotherapy.- Protein kinases and multidrug resistance.- New insights into the kinetic resistance to anticancer agents.- The extended-MDR phenotype.- Role of oxygenation and vascularization in drug resistance.- Transcriptional regulation of MDR genes.- Cytokine-mediated reversal of multidrug rsistance.- The role of oncogene in drug resistance.- Apoptosis resistance in tumor cells.- Regulation of caspase activation in apoptosis: implications for transformation and drug resistance.- Regulation of keratin and integrin gene expression in cancer and drug resistance.Introduction
Martin Clynes