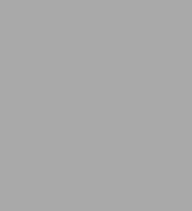
Nanochemistry: A Chemical Approach to Nanomaterials
820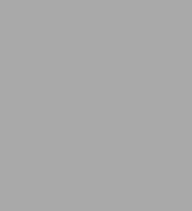
Nanochemistry: A Chemical Approach to Nanomaterials
820eBook
Available on Compatible NOOK devices, the free NOOK App and in My Digital Library.
Related collections and offers
Overview
Product Details
ISBN-13: | 9781782626268 |
---|---|
Publisher: | Royal Society of Chemistry |
Publication date: | 10/09/2015 |
Sold by: | Barnes & Noble |
Format: | eBook |
Pages: | 820 |
File size: | 52 MB |
Note: | This product may take a few minutes to download. |
About the Author
Geoffrey A. Ozin obtained his undergraduate degree at Kings College London and his graduate degree at Oriel College Oxford. Following post-doctoral research as an ICI Fellow at Southampton University he joined the University of Toronto where he is now Government of Canada Research Chair in Materials Chemistry and University Professor.
He is also Honorary Professor at The Royal Institution of Great Britain and University College London, as well as a Member of the London Centre for Nanotechnology.
André C. Arsenault is Chief Technology Officer and cofounder of Opalux Inc., a Toronto-based company developing products based on opal technology. He completed his honours degree in Biological Chemistry at the University of Toronto in 2001 followed by a PhD in the groups of Geoffrey A. Ozin and Ian Manners in 2006. He is currently the author of 21 scientific publications, and the holder of one US patent. His work has appeared several times in the news media.
Ludovico Cademartiri is a PhD student in the group of Geoffrey A. Ozin at the University of Toronto. He completed his Laurea cum laude in Materials Science at the University of Parma, Italy in 2002 before joining the graduate program in interdisciplinary chemistry at the University of Toronto. He is the author of 12 scientific publications and has been awarded the CRC Graduate Prize in Chemistry and the CSC DIC Prize for Graduate Work in Inorganic Chemistry.
Read an Excerpt
Nanochemistry A Chemical Approach to Nanomaterials
By Geoffrey A. Ozin, André C. Arsenault, Ludovico Cademartiri
The Royal Society of Chemistry
Copyright © 2009 The Royal Society of ChemistryAll rights reserved.
ISBN: 978-1-78262-626-8
CHAPTER 1
Nanochemistry Basics
"We are like dwarfs on the shoulders of giants, so that we can see more than they."
Bernard of Chartres, 12th century
1.1 The Roots of Nanochemistry in Materials Chemistry
Since one of us began teaching materials chemistry in the late sixties, the field has undergone revolutionary developments. The subject had never before been part of the undergraduate or graduate degree program in our university chemistry department. At this time one could obtain a degree in materials science and engineering but the chemistry content of the courses was negligible and teaching the rudiments of the chemical synthesis of materials seemed to be of minor importance. I felt this was odd considering synthesis is where the solid state food chain begins and the paradigm of relating structure to property to function and utility emerges from studies of the X-ray diffraction structure, electronic band properties and defects in solids, knowledge of which leads to utility of solids in advanced materials technologies.
My impression of the way the world of materials worked at that time was that traditional materials scientists are not the ones who dream up novel synthetic pathways to new materials and they are actually not that interested in the chemical properties of materials. Indeed, materials scientists are renowned for understanding the mechanical properties of materials and engineering materials into products, processes and devices but they are not really recognized for imagining new ways of preparing novel classes of materials from scratch. Instead, they tend to modify and improve existing materials to achieve a specific set of properties, a targeted function and a practical application. It is clear that such distinctions are bound to blur as materials science and engineering departments are increasingly hiring chemists to increase their "scientific footprint" in nanoscience.
My proposal for teaching materials chemistry was adopted, and teaching the subject for the first time to undergraduate and graduate students enabled me to build a broad and deep appreciation of the field of solid state materials chemistry. It made me realize the importance of interdisciplinary teaching and research facilitated by crossing the boundaries between chemistry, physics, materials science and engineering, biology and medicine, which helped my teaching of materials chemistry to chemistry students with the added benefit that it allowed me to develop original ideas important for research. It also put me in good stead for teaching and research in the multidisciplinary field of nanoscience, and helped me to write the book you are now reading.
I contend in fact that materials chemistry lies at the heart of nanochemistry and that knowledge of the bulk synthesis techniques which have been exhaustively developed in the past century is greatly advantageous in approaching nanomaterials. In most cases the synthesis routes to nanomaterials are adaptations of well known bulk material syntheses. We even suggest here that nanochemistry might become a tool to better understand some aspects of materials chemistry which we couldn't previously deal with. We are here aiming to the synthesis of materials on a length scale and with surface chemistries that can modify several chemical processes, like nucleation and growth.
The approach to teaching materials chemistry that I prefer is mainly through the synthesis of materials, I attempt to offer an umbrella view of the field by systematically looking at all classes of materials and all the ways they are made in bulk formats, and then focus on their structure and properties, functions and potential applications. With this knowledge I then proceed to ask how they can be down-sized through chemistry to the nanoscale format. What I mean by nanoscale format is solid state building blocks with particular composition and structure but with controlled size, shape and surface functionality, the theme running through this entire text.
When thinking about nanomaterials one realizes they are often reconstructions of known materials in bulk form. So what is new about nanomaterials? Their physical size is approximately in the range between 1nm and 1000 nm; their chemical and physical properties scale in predictable ways with size; synthetic strategies are designed to create them with strict control over size, shape and surface properties; self-assembly procedures are used to organize and build them into functional architectures with a purpose.
So nanomaterials can be viewed as reconstructed forms of well-known bulk materials like superconductors and metals, semiconductors and ceramics, polymers and biopolymers. They may have similar compositions and structures to their bulk analogues but nanomaterials are sculpted into a particular shape, bounded by a much smaller volume, and fashioned with a much higher surface area, and as a result exhibit distinctive behavior.
In the field of nanomaterials it is not just discovering a new composition or structure that is challenging but also dreaming up effective ways of rebuilding known materials into desired nanoscale building blocks. Actually, it is quite hard to think of a nanomaterial that does not also exist in the bulk form or is at least closely related to it. Because of the composition-structure relationship between most bulk and nanoscale forms of matter one finds that synthetic methods to downsize materials fall into two main categories, the first involves creative modification of a known synthesis for making a bulk material to target the nanomaterial analogue while the second requires the discovery of entirely new synthetic pathways to the desired nanomaterial.
1.2 Synthesis of Materials and Nanomaterials
The following chapters are mainly about how to synthesize diverse classes of size, shape and surface controlled nanomaterials with properties that evoke a specific function and can be intentionally tailored for a particular use. In presenting the subject it is assumed that the reader has a basic understanding of classes and structures of solids, states of matter, electronic bands, defects and non-stoichiometry, electronic and ionic transport.
This fundamental knowledge allows one to appreciate why different classes of solids exhibit particular properties like ferromagnetism, piezoelectricity, superconductivity, photorefractivity, electrochromism, thermoelectricity, that make them useful for different applications. How scale affects the properties of materials is a central theme of nanoscience and how this knowledge can be usefully applied is the basis of future nanotechnology.
When thinking about going into the laboratory to actually synthesize a specific nanomaterial it is useful to first recall how the bulk material is made and whether that method can be successfully adapted to yield the same material but at the nanoscale. If this route does not seem practical then one has to devise a new pathway that will achieve the objective.
To amplify on this point, presented below is a synopsis of the most common ways of synthesizing solid-state materials in bulk format. From here, we can explore the challenges of how to modify these methods or invent new approaches that allow nanomaterials to be synthesized with control over size, shape and surface:
Direct reaction chemistry
[MATHEMATICAL EXPRESSION OMITTED]
Solution phase precursors are mixed in the exact molar ratios and thermally treated to create the desired solid state product with the right stoichiometry, in this case a compositionally controlled magnetic Garnet.
Single source precursor chemistry
[MATHEMATICAL EXPRESSION OMITTED]
Barium bis-oxalatotitanyl(IV) coordination solid, containing barium and titanium in the right proportion and oxidation states is thermally treated to make barium titanate, a Perovskite with interesting ferroelectric and photorefractive properties.
Rapid solid state metathesis exchange chemistry
[MATHEMATICAL EXPRESSION OMITTED]
The highly exothermic reaction between solid precursors, driven mainly by the large heat of formation of sodium chloride product, ignites and self-propagates the metathesis exchange to form molybdenum disulfide.
Vapor phase transport chemistry
[MATHEMATICAL EXPRESSION OMITTED]
The direct reaction between chromium sesquioxide and nickel oxide solid reagents to form nickel chromite Spinel at high temperature is slow and incomplete but in the presence of oxygen gas (transport agent), less reactive chromium sesquioxide solid is converted to highly reactive chromium trioxide vapor, which diffuses to, and reacts with the nickel oxide at a higher rate and to give a better yield of product Spinel.
Chemical vapor deposition chemistry
[MATHEMATICAL EXPRESSION OMITTED]
Vapors of trimethylgallium and arsine co-adsorb on a hot substrate and react to form a film of semiconductor gallium arsenide where the methyl groups are removed by the carrier gas hydrogen.
Ion-exchange chemistry
[MATHEMATICAL EXPRESSION OMITTED]
The charge balancing sodium ions that reside in the conduction plane of the beta- alumina defect Spinel are exchanged for neodymium cations (three sodium(I) ions for each neodymium(III) ion) by immersing the Spinel in a stoichiometric melt of neodymium trichloride dissolved in sodium chloride.
Intercalation chemistry
[MATHEMATICAL EXPRESSION OMITTED]
The stoichiometric reaction of a solution of butyl lithium in tetrahydrofuran with solid titanium disulfide results in the insertion of lithium ions into the van der Waals gap between the layers of titanium disulfide and concomitant placement of charge balancing electrons into the titanium disulfide conduction band.
Host-guest inclusion chemistry
[MATHEMATICAL EXPRESSION OMITTED]
When disilane gas is exposed to the solid acid form of zeolite Y, the disilane reacts with protons in the supercage to form anchored disilyl groups that subsequently reductively eliminate hydrogen and agglomerate within the supercage of zeolite Y to form encapsulated photoluminescent silicon nanoclusters.
Sol-gel chemistry
[MATHEMATICAL EXPRESSION OMITTED]
Hydrolytic poly-condensation of a stoichiometric mixture of tributoxyindium(III) and tetrabutoxytin(IV) in a ratio of about 10:1, followed by thermal post-treatment of the gel so-formed, yields p-doped indium tin oxide (ITO)
Chimie douce
[MATHEMATICAL EXPRESSION OMITTED]
Literal translation soft-chemistry, the Rutile polymorph of titanium dioxide when reacted with potassium oxide is converted to a complex layered form of titanium oxide that on conversion to the acidic gel form can be thermally transformed back to titanium dioxide but retaining the layered structure of the gel rather than the Rutile structure.
Electrochemical synthesis
[MATHEMATICAL EXPRESSION OMITTED]
The electrochemical oxidation of an aluminum anode in concentrated phosphoric acid electrolyte using a platinum working electrode, involves the production of Al3+ions with concomitant reduction of phosphate to phosphite ions plus co-generated oxide O2- ions, where the oxide ions react with the aluminum ions to form an over coating of aluminum trioxide on the aluminum (note that oxide and aluminum ion reactants have to diffuse through an ever thickening aluminum trioxide product layer as the anodic oxidation proceeds).
Hydrothermal chemistry
[MATHEMATICAL EXPRESSION OMITTED]
Aqueous aluminate and silicate precursors in the presence of base undergo hydrolytic poly-condensation to form a sodium aluminosilicate gel that under hydrothermal reaction conditions crystallizes into a microporous sodium aluminosilicate zeolite with water imbibed within the micropores.
Chemistry at high pressures and temperatures
[MATHEMATICAL EXPRESSION OMITTED]
Pressurizing solid C60 at 20 GPa in a high pressure diamond anvil reactor causes it to rapidly transform to bulk crystalline diamond, a process that is facilitated by the intermediate sp2-3 hybridization of C60 between graphite and sp2 and diamond and sp3.
The goal of the synthesis methods summarized above is to create solid state compositions with intentionally designed structure and properties, form and function aimed at a particular technology, like the hydrothermal synthesis of large crystals of quartz used in piezoelectric oscillators. It should be kept in mind that because many of the syntheses shown above involve direct reaction between solid state precursors the rate of the reaction and product yield can both be enhanced by minimizing the diffusion lengths of species participating in the reactions. Interestingly, this can often be achieved by reducing the physical dimensions of reagents to the nanoscale, indicating the clear cut reactivity advantage of nanochemistry.
In closing one should note that when synthesizing nanomaterials it may be possible to modify one of the traditional solid state synthesis procedures described above, such that instead of yielding bulk material in the form of spheres, rods or plates, the method creates the material as nanoclusters, nanowires or nanosheets. If this does not prove possible in practice then an alternative route will have to be sought in order to achieve the objective. This is the essence of shape controlled synthesis of nanomaterials delineated in the chapters that follow.
1.3 Materials Self-Assembly
When thinking about self-assembly of a targeted structure from the spontaneous organization of building blocks with dimensions that are beyond the sub-nanometer scale of most molecules or macromolecules, there are five prominent principles that need to be taken into consideration. These are: (i) building blocks, scale, shape, surface structure, (ii) attractive and repulsive interactions between building blocks, equilibrium separation, (iii) reversible association-dissociation and/or adaptable motion of building blocks in assembly, lowest energy structure, (iv) building block interactions with solvents, interfaces, templates, (v) building-block dynamics, mass transport and agitation.
A challenge for perfecting structures made by this kind of self-assembly chemistry is to find ways of synthesizing (bottom-up) or fabricating (top-down) building blocks not only with the right composition but also having the same size and shape. No matter which way building blocks are made they are never truly monodisperse, unless they happen to be single atoms, molecules or clusters. There exists a degree of polydispersity in their size and shape, which is manifest in the achievable degree of structural perfection of the assembly and the nature and population of defects in the assembled system. Equally demanding is to make building blocks with a particular surface structure, charge and functionality. Surface properties will control the interactions between building blocks as well as with their environment, which ultimately determines the geometry and distances at which building blocks come to equilibrium in a self-assembled system. Relative motion between building blocks facilitates collisions between them, whilst energetically allowed aggregation and de-aggregation processes and corrective movements of the self-assembled structure will allow it to attain the most stable form. Providing the building blocks are not too strongly bound in the assembly they will be able to adjust to an orderly structure. If on the other hand the building blocks in the assembly interact too strongly, they will be unable to adjust their relative positions within the assembly and a less ordered metastable structure will result. Dynamic effects involving building blocks and assemblies can occur in the liquid phase, at an air/liquid or liquid/liquid interface, on the surface of a substrate or within a template co-assembly.
As this text describes, building blocks can be made out of most known organic, inorganic, polymeric, and hybrid materials. Creative ways of making spheres and cubes, sheets and discs, wires and tubes, rings and spirals, with nm to cm dimensions, abound in the materials self-assembly literature. They provide the basic construction modules for materials self-assembly over all scales, a new way of synthesizing electronic, optical, photonic, magnetic materials with hierarchical structures and complex form, which is the central theme running throughout this chapter. A flowchart describing these main ideas is shown in Figure 1.1.
(Continues...)
Excerpted from Nanochemistry A Chemical Approach to Nanomaterials by Geoffrey A. Ozin, André C. Arsenault, Ludovico Cademartiri. Copyright © 2009 The Royal Society of Chemistry. Excerpted by permission of The Royal Society of Chemistry.
All rights reserved. No part of this excerpt may be reproduced or reprinted without permission in writing from the publisher.
Excerpts are provided by Dial-A-Book Inc. solely for the personal use of visitors to this web site.