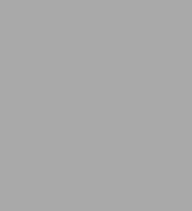
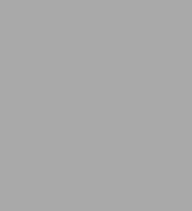
Paperback(Reprint)
-
PICK UP IN STORECheck Availability at Nearby Stores
Available within 2 business hours
Related collections and offers
Overview
But as Ronald T. Merrill reveals in Our Magnetic Earth, geomagnetism really is an enduring, vibrant area of science, one that offers answers to some of the biggest questions about our planet’s pastand maybe even its future. In a clear and careful fashion, he lays out the physics of geomagnetism and magnetic fields, then goes on to explain how Earth’s magnetic field provides crucial evidence for our understanding of continental drift and plate tectonics; how and why animals, ranging from bacteria to mammals, sense and use the magnetic field; how changes in climate over eons can be studied through variations in the magnetic field in rocks; and much more. Throughout, Merrill peppers his scientific account with bizarre anecdotes and fascinating details, from levitating pizzas to Moon missions to blackmailing KGB agentsa reminder that real science can at times be stranger, and more amusing, than fiction.
A winning primer for anyone who has ever struggled with a compass or admired a ragged V of migrating geese, Our Magnetic Earth demonstrates that education and entertainment need not be polar opposites.
Product Details
ISBN-13: | 9780226006598 |
---|---|
Publisher: | University of Chicago Press |
Publication date: | 10/03/2012 |
Edition description: | Reprint |
Pages: | 272 |
Product dimensions: | 6.00(w) x 8.90(h) x 0.90(d) |
About the Author
Read an Excerpt
Our Magnetic Earth
The Science of GeomagnetismBy RONALD T. MERRILL
THE UNIVERSITY OF CHICAGO PRESS
Copyright © 2010 The University of ChicagoAll right reserved.
ISBN: 978-0-226-52050-6
Chapter One
Magnetism and the Present Magnetic Field
If there had been a human with a compass 800,000 years ago, he would have found his compass needle pointed south rather than north. Twenty thousand years later Earth's magnetic field reversed. The magnetic north and south poles swapped hemispheres. The compass needle also would have flipped. Reversals have occurred hundreds of times in Earth's history. Our magnetic field is constantly changing, even during our lifetimes. During the twentieth century, the strength of the field declined by 6 percent, and the magnetic north pole moved northward by nearly 10° in latitude.
What causes our magnetic field to be so restless? What are the consequences of this restlessness? Do variations in Earth's magnetic field relate to magnetic storms that sometimes cause major power outages? Will they affect our climate? Will animals that sense, and use, the magnetic field encounter serious problems? These are just a few of the questions I have been asked by students and professional scientists. As a professor specializing in geomagnetism (the study of Earth's magnetic field) at the University of Washington, the questioners thought I would always be able to provide definitive answers. Sometimes I could and sometimes not.
In this book, I cover a broad range of topics dealing with magnetism in the earth and planetary sciences. I also provide you with the reasons why geomagnetists arrive at their conclusions. I will do this without using the mathematical language that we geomagnetists often use. To make our journey in geomagnetism more alive, I will provide some historical context and introduce you to some of the characters I have met during my career.
In this chapter, magnetism and magnetic fields are treated first, as they are at the foundations of geomagnetism. Then our knowledge of the present field is developed in a historical context.
Our bewilderment and fascination with magnetism often began when we were introduced to magnets as children. We found it strange that two bar magnets in one configuration resisted being pushed together, while in the opposite one they attracted each other. Why? In my case, I felt the need to consult someone of authority. I turned to my father, who as a parent surely must know the correct answer. I was fortunate, because as an authority goes, he had the correct credentials—a PhD in theoretical physics. He explained to me that there are two ends to a magnet, a plus (or north) end and a minus (or south) end. When the two ends facing each other have the same sign, they repel each other. When they have opposite signs, they attract. He repeated this explanation a few different times to make sure I understood. Many of you will have received a similar explanation from an "authority," perhaps a high school science teacher. What was your reaction to your authority's explanation? I remember my reaction to my father's explanation: I must be dumb. I could see the magnets were attracted to each other in one alignment and were repelled in the opposite one, but I wanted to know why. I thought my authority must have given me the correct explanation, but I was simply not smart enough to understand it. I will say more about his answer and authority arguments as we travel through this book.
History illustrates that I was not alone in being puzzled by the seemingly mysterious properties of magnets. I suspect that humans have been interested in the origin of magnetism ever since it became recognized that some minerals were magnetic. Magnetism was investigated over 2,000 years ago in two separate political systems, one centered on the Mediterranean Sea and one centered in China. These early studies focused on lodestone, which is more commonly known today as the mineral magnetite, an iron oxide (Fe3O4). It was mined in the province of Magnesia (Thessaly, Greece), and it became known by the Latin word magneta, from which the modern term "magnet" derives.
William Gilbert of Colchester (1544–1603), often referred to as the father of magnetism for his reliance on the (now-called) experimental method, attributed the attractive powers of lodestone to it having a soul, similar to the views of the Greek animist Anaxagoras around 460 BC. Gilbert's most important legacy came with the publication of De Magnete in 1600. In it, he reproduced many of the results of Petrus Peregrinus, whose remarkable experiments with spheres of lodestone in 1 69 emphasized the dipolar nature of magnets: they always come with two poles, a plus one and a minus one. Gilbert recognized that Peregrinus's spheres could be viewed as models of Earth. Gilbert pointed out that "rays of magnetick virtue" spread out in every direction in an "orbe." The center of this orb is not at the pole, as had been conjectured by Peregrinus and others, but in the center of the stone. Building on this, Gilbert was the first to conclude that Earth itself behaves like a huge magnet. Gilbert also noticed that Earth's magnetic and geographic poles were in close proximity, arguing that this showed Earth's rotation about its axis stemmed from its magnetism. Later the philosopher René Descartes (1596–1650) exorcised the soul from lodestone and embraced Gilbert's view that Earth could be viewed as a large magnet.
Today we believe the main magnetic field originates from electric currents flowing in Earth's iron-rich core. (The process by which these currents are generated is the so-called dynamo, a concept that will be discussed in more detail later.) Gilbert and others could not have arrived at such a conclusion in their time because scientists were not aware of electric currents until the studies of Stephen Gray (1666–1736). The connection between electric currents and magnetism was not recognized until much later by Michael Faraday (1791–1867) and Hans Christian Oersted (1777–1851). In Gilbert's day, scientists were unable to construct the theory we accept today for the origin of Earth's magnetic field because the crucial physics had not yet been discovered. They had little recourse but to attribute the origin of the magnetic field to permanent magnetism. It makes one pause and wonder what incorrect explanations we hold today because we have not yet developed the crucial underlying science.
* * *
Even today it is sometimes difficult to know whether a magnetic field has arisen from electric currents or from magnetization, as I learned while serving on NASA's Lunar and Planetary Science Review Panel from 1978 through 1980. Well before the manned (Apollo) missions brought back lunar samples, potential astronauts had been trained in the southwestern United States to map rocks in the same way geologists did on Earth. At that time geologists often used a pace and compass technique: pacing to estimate distance and a magnetic compass to estimate direction. This training went on for several months until someone pointed out that the Moon had no magnetic field, which makes it impossible to rely on a compass for direction. When the first lunar samples were later returned to Earth, scientists were shocked to find some of them were magnetized. How could they be magnetized without the Moon having a magnetic field? Some scientists thought an error must have occurred. NASA even arranged to have a lunar sample sent back to the Moon and returned to Earth to make sure the magnetization was not accidentally acquired during transit. Over time, further missions showed that many lunar rocks were magnetized, and magnetic field measurements from various spacecraft showed that the Moon has a weak magnetic field stemming from a magnetized crust. The lunar magnetic field is complex, exhibiting many magnetic poles (where the field is vertical). Because the lunar magnetic field is weak and complex, magnetic compasses would be essentially useless on the Moon. It remains a mystery as to how the lunar crust became magnetized. Our best guess, and it really is little more than a guess, is the Moon had a dynamo-produced field for its first half billion years or so, as does Earth today (chap. 3).
We can gain more insight into magnetic fields by considering a different field, that of gravity. Like the magnetic force, the gravitational force acts at a distance. When I was a child, it did not seem as strange to me as did the magnetic force. Perhaps this is because we are constantly experiencing the gravitational force and even measuring it—one's weight is a measure of the gravitational force. A force is a push or pull specified by a direction and a magnitude. The gravitational force exerted by Earth is downward toward Earth's center, not sidewise or upward. The magnitude of the gravitational force between two objects is proportional to the product of their masses divided by the square of the distance. If the distance between two objects is doubled, the force decreases by a factor of four. After many measurements were made to confirm this, scientists accepted that they had a "law of gravity," first mathematically formulated by Sir Isaac Newton in 1687. Although tests of this law indicate that it is an excellent one, we have not answered the question why does this law exist? We simply have observed that it does, and like our best laws, Newton constructed a mathematical relation allowing us to make accurate predictions.
My wife and I weigh different amounts. That is, the gravitational force of Earth acting on my wife is different from that acting on me. Yet we would like to describe Earth's gravity at a particular location by a single number. This would allow us to do many things, including making comparisons of Earth's gravity to that of other planets. Earth's gravity is, for example, different from the Moon's gravity; we weigh about one-sixth as much on the Moon as on Earth. The concept of a gravitational field allows us to do this. One can determine the gravitational field of Earth by dividing the gravitational force by the mass it attracts. In other words, the gravitational field is the gravitational force per unit mass. This means Earth's gravitational field depends only on the mass of Earth and not the mass of the object Earth is attracting. If we want to know the gravitational force acting on, say, my wife, we can obtain this by multiplying her mass by the gravitational field.
A similar definition can be used for the electric force between two electric charges, which is proportional to the product of the charges divided by the square of the distance between them. The electric field is defined as the electric force on a unit of positive charge (such as that of a proton). The electric field does not depend on the magnitude of the charge that the electric field acts upon.
Scientists define a magnetic field in an analogous way: it is the force on a unit of positive magnetic charge. But there is a catch. No one has found an isolated magnetic charge, a magnetic monopole. Scientists have never even found indirect evidence that a single "magnetic charge" exists, unlike that in electricity where it is well documented that an electron has a single negative charge (an electric monopole) and a proton has a single positive charge. Nevertheless, the Nobel Prize–winning physicist Paul Dirac (190 –1984) postulated the existence of magnetic monopoles based on symmetry arguments. In particular, Dirac demonstrated that the famous Maxwell equations could be modified to include magnetic monopoles.
The equations modified by Dirac were four partial differential equations developed by James Clerk Maxwell (1831–1879) that unified electricity and magnetism. Rather than speaking of separate electric and magnetic fields, one could speak of an electromagnetic field. When electric charges move to produce an electric current, a magnetic field is simultaneously produced. Maxwell even used these equations to show that light traveled as an electromagnetic wave. More than one advanced course (graduate-student level) in the twenty-first century began by the professor writing the Maxwell equations down on the board and proclaiming that is all there is to electricity and magnetism—only the details require explaining, which will take the remainder of the year. While an exaggeration, such a statement illustrates the central role that the Maxwell equations play in electricity and magnetism.
Dirac showed that a simple modification to Maxwell's equations produced a symmetry between electricity and magnetism. Just as electric currents produce magnetic fields, the modified Maxwell equations permit magnetic currents, which produce electric fields. Although symmetry arguments are powerful instruments for advancing physics, so far no one has demonstrated that a magnetic monopole exists. But scientists are still searching for one!
You might wonder, if there are no known monopoles, what produces the magnetic field of magnets? Consider a simple bar magnet—a magnet with a length much greater than its width. Suppose we decide to cut this magnet into two parts in an attempt to separate the plus end from the minus end. This would result in two magnets, each with its own plus and minus ends. In principle, we could repeat this experiment as often as we like. But if we did, we would always obtain magnets with two poles, a plus and a minus one. We could continue this thought experiment until we reduced the magnet's size down to a single electron. The electron is a fundamental particle, and further subdivision appears impossible. The electron still has two poles and behaves like a magnet. We refer to this magnet as a "point dipole," or simply "dipole." The word "dipole" refers to the fact that there are two poles—a plus one and a minus one. It is sufficient for us to accept as an observational fact that there is a magnetic dipole field, which is experimentally found to be tied to the electron's spin. Just as a moving electron produces a magnetic field, so does a rotating (spinning) electron.
Unlike a monopole field (for example, the electric field from an electron), the magnitude of a dipole field falls off as the cube of the distance from the source. If the distance between the point where the field is measured and the dipole's position is doubled, the magnitude of the field is reduced by a factor of 1/8. Because a dipole consists of plus and negative magnetic charges, it both repels and attracts any other magnetic charge. Because of these opposing effects, the magnetic field from a dipole falls off faster with distance than it would if only one magnetic charge were present.
The sources of Earth's magnetic field cannot be uniquely determined from measurements made at or above Earth's surface, a point I will return to later in this chapter. Although this causes us some difficulties in explaining the origin of Earth's magnetic field (as we shall see in chapter 3), it allows us to represent much of Earth's magnetic field by the geocentric axial dipole field shown in figure 1.1. This dipole (which can be visualized as a bar magnet) is at Earth's center and aligned along Earth's rotation axis. We represent the field this way, even though the actual magnetic field does not originate from a strong dipole at Earth's center. We do this because it is mathematically convenient and because it is easy to visualize. This geocentric axial dipole field is vertical at two points: the geomagnetic north and south poles. These two points coincide with the geographic poles because the field is along the rotation axis. The field lines are also shown. While these lines are not real physical entities, they are useful devices to describe the direction and magnitude of the magnetic field at any locality.
(Continues...)
Excerpted from Our Magnetic Earth by RONALD T. MERRILL Copyright © 2010 by The University of Chicago. Excerpted by permission of THE UNIVERSITY OF CHICAGO PRESS. All rights reserved. No part of this excerpt may be reproduced or reprinted without permission in writing from the publisher.
Excerpts are provided by Dial-A-Book Inc. solely for the personal use of visitors to this web site.
Table of Contents
AcknowledgmentsChapter 1. Magnetism and the Present Magnetic Field
Chapter 2. Magnetic Field Reversals
Chapter 3. Earth’s Internal Composition and the Origin of Earth’s Magnetic Field
Chapter 4. The Sun-Earth Connection
Chapter 5. Magnetic Orientation and Navigation by Animals
Chapter 6. The Effects of Geomagnetism and Plate Tectonics on Climate and Paleoclimate
Epigraph Some Parting Comments
Appendix: Rock Magnetism Fundamentals
Notes
Index