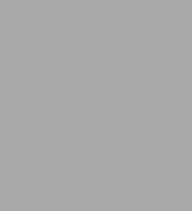
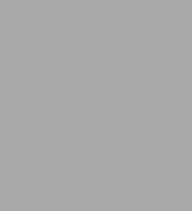
eBook
Available on Compatible NOOK devices, the free NOOK App and in My Digital Library.
Related collections and offers
Overview
Physicochemical and Environmental Plant Physiology, Fourth Edition, is the updated version of an established and successful reference for plant scientists. The author has taken into consideration extensive reviews performed by colleagues and students who have touted this book as the ultimate reference for research and learning.
The original structure and philosophy of the book continue in this new edition, providing a genuine synthesis of modern physicochemical and physiological thinking, while entirely updating the detailed content. This version contains more than 40% new coverage; five brand new equations and four new tables, with updates to 24 equations and six tables; and 30 new figures have been added with more than three-quarters of figures and legends improved. Key concepts in plant physiology are developed with the use of chemistry, physics, and mathematics fundamentals.
The book is organized so that a student has easy access to locate any biophysical phenomenon in which he or she is interested.
- More than 40% new coverage
- Incorporates student-recommended changes from the previous edition Five brand new equations and four new tables, with updates to 24 equations and six tables 30 new figures added with more than three-quarters of figures and legends improved Organized so that a student has easy access to locate any biophysical phenomenon in which he or she is interested Per-chapter key equation tables Problems with solutions presented in the back of the book Appendices with conversion factors, constants/coefficients, abbreviations and symbols
Product Details
ISBN-13: | 9780080920894 |
---|---|
Publisher: | Elsevier Science |
Publication date: | 05/13/2009 |
Sold by: | Barnes & Noble |
Format: | eBook |
Pages: | 604 |
File size: | 11 MB |
Note: | This product may take a few minutes to download. |
About the Author
He eventually shifted toward plant physiological ecology and has written six books on the subject that have been cited extensively. Besides writing these texts, he has published six books on agaves and cacti. He has also authored nearly 400 scientific research articles and reviews.
Dr. Nobel has developed original equations for the air boundary layers surrounding cylinders and spheres and has championed the importance of the mesophyll surface area per unit leaf area, Ames/A. Other research topics have included the importance of shallow root distribution for taking advantage of light desert rainfalls and the influences of an air gap developing around roots during drought on root-soil water movement.
Read an Excerpt
Physicochemical and Environmental
Plant PhysiologyBy Park S. Nobel
Academic Press
Copyright © 2009 Elsevier Inc.All right reserved.
ISBN: 978-0-08-092089-4
Chapter One
Cells and Diffusion
1.1. Cell Structure 3 1.1A. Generalized Plant Cell 3 1.1B. Leaf Anatomy 5 1.1C. Vascular Tissue 7 1.1D. Root Anatomy 9
1.2. Diffusion 11 1.2A. Fick's First Law 12 1.2B. Continuity Equation and Fick's Second Law 14 1.2C. Time–Distance Relation for Diffusion 16 1.2D. Diffusion in Air 19
1.3. Membrane Structure 21 1.3A. Membrane Models 21 1.3B. Organelle Membranes 23
1.4. Membrane Permeability 25 1.4A. Concentration Difference Across a Membrane 26 1.4B. Permeability Coefficient 28 1.4C. Diffusion and Cellular Concentration 29
1.5. Cell Walls 31 1.5A. Chemistry and Morphology 33 1.5B. Diffusion Across Cell Walls 34 1.5C. Stress–Strain Relations of Cell Walls 37 1.5D. Elastic Modulus, Viscoelasticity 39
1.6. Problems 40
1.7. References and Further Reading 42
1.1. Cell Structure
Before formally considering diffusion and related topics, we will outline the structures of certain plant cells and tissues, thus introducing most of the anatomical terms used throughout this book.
1.1A. Generalized Plant Cell
Figure 1-1 depicts a representative leaf cell from a higher plant and illustrates the larger subcellular structures. The living material of a cell, known as the protoplast, is surrounded by the cell wall. The cell wall is composed of cellulose and other polysaccharides, which helps provide rigidity to individual cells as well as to the whole plant. The cell wall contains numerous relatively large interstices, so it is not the main permeability barrier to the entry of water or small solutes into plant cells. The main barrier, known as the plasma membrane (or plasmalemma), is found inside the cell wall and surrounds the cytoplasm. The permeability of this membrane varies with the particular solute, so the plasma membrane can regulate what enters and leaves a plant cell. The cytoplasm contains organelles such as chloroplasts and mitochondria, which are membrane-surrounded compartments in which energy can be converted from one form to another. Chloroplasts, whose production and maintenance is a primary function of plants, are the sites for photosynthesis, and mitochondria are the sites for respiration. Microbodies, such as peroxisomes and ribosomes, are also found in the cytoplasm along with macromolecules and other structures that influence the thermodynamic properties of water. Thus, the term cytoplasm includes the organelles (but generally not the nucleus), whereas the term cytosol refers to the cytoplasmic solution delimited by the plasma membrane and the tonoplast (to be discussed next) but exterior to the organelles.
In mature cells of higher (evolutionarily advanced) plants and many lower plants, there is a large central aqueous compartment, the central vacuole, which is surrounded by a membrane called the tonoplast. The central vacuole is usually quite large and can occupy up to about 90% of the volume of a mature cell. Because of the large central vacuole, the cytoplasm occupies a thin layer around the periphery of a plant cell (Fig. 1-1). Therefore, for its volume, the cytoplasm has a relatively large surface area across which diffusion can occur. The aqueous solution in the central vacuole contains mainly inorganic ions or organic acids as solutes, although considerable amounts of sugars and amino acids may be present in some species. Water uptake by this central vacuole occurs during cell growth and helps lead to the support of a plant.
One immediate impression of plant cells is the great prevalence of membranes. In addition to surrounding the cytoplasm, membranes also separate various compartments in the cytoplasm. Diffusion of substances across these membranes is much more difficult than is diffusion within the compartments. Thus, organelle and vacuolar membranes can control the contents and consequently the reactions occurring in the particular compartments that they surround. Diffusion can also impose limitations on the overall size of a cell because the time for diffusion can increase with the square of the distance, as we will quantitatively consider in the next section.
Although many plant and algal cells share most of the features indicated in Figure 1-1, they are remarkably diverse in size. The cells of the green alga Chlorella are approximately 4 x 10-6m (4 µm) in diameter. In contrast, some species of the intertidal green alga Valonia have multinucleated cells as large as 20 mm in diameter. The genera Chara and Nitella include fresh-and brackish-water green algae having large internodal cells (Fig. 3-13) that may be 100 mm long and 1 mm in diameter. Such large algal cells have proved extremely useful for studying ion fluxes, as we consider in Chapter 3 (e.g., Sections 3.2E; 3.3E,F).
1.1B. Leaf Anatomy
A cross section of a typical angiosperm (seed plant) leaf can illustrate various cell types and anatomical features that are important for photosynthesis and transpiration. Leaves are generally 4 to 10 cells thick, which corresponds to a few hundred micrometers (Fig. 1-2). An epidermis occurs on both the upper and the lower sides of a leaf and is usually one cell layer thick. Except for the guard cells, epidermal cells usually are colorless because their cytoplasm contains few, if any, chloroplasts (depending on the species). Epidermal cells have a relatively thick waterproof cuticle on the atmospheric side (Fig. 1-2). The cuticle contains cutin, which consists of a diverse group of complex polymers composed principally of esters of 16- and 18-carbon monocarboxylic acids that have two or three hydroxyl groups (esterification refers to the chemical joining of an acid and an alcohol resulting in the removal of a water molecule). Cutin is relatively inert and also resists enzymatic degradation by microorganisms, so it is often well preserved in fossil material. We will consider its role in minimizing water loss from a leaf.
Between the two epidermal layers is the mesophyll (literally, middle of the leaf) tissue, which is usually differentiated into chloroplast-containing "palisade" and "spongy" cells. The palisade cells are often elongated perpendicular to the upper epidermis and are found immediately beneath it (Fig. 1-2). The spongy mesophyll cells, located between the palisade mesophyll cells and the lower epidermis, are loosely packed, and intercellular air spaces are conspicuous. In fact, most of the surface area of both spongy and palisade mesophyll cells is exposed to air in the intercellular spaces, facilitating diffusion of gases into or out of the cells. A spongy mesophyll cell is often rather spherical, about 20 µm in radius, and can contain approximately 40 chloroplasts. (As Fig. 1-2 illustrates, the cells are by no means geometrically regular, so dimensions here indicate only approximate size.) A neighboring palisade cell is usually more oblong; it can be 80 µm long, can contain 60 chloroplasts, and might be represented by a cylinder 15 µm in radius and 50 µm long with hemispherical ends. Based on the dimensions given, a spongy mesophyll cell can have a volume of
V = (4/3)(π)(20 x 10-6 m)3 = 3.4 x 10-14 m3
A palisade mesophyll cell can have a volume of
V = (π)(15 x 10-6 m)2(50 x 10-6 m) + (4/3)(π)(15 x 10-6 m)3 = 4.9 x 10-14m3
(formulas for areas and volumes of various geometric shapes are given in Appendix IIIB.) In many leaves, palisade mesophyll cells contain about 70% of the chloroplasts and often outnumber the spongy mesophyll cells nearly two to one.
The pathway of least resistance for gases to cross an epidermis–and thus to enter or to exit from a leaf–is through the adjustable space between a pair of guard cells (Fig. 1-2). This pore, and its two surrounding guard cells, is called a stoma or stomate (plural: stomata and stomates, respectively). When they are open, the stomatal pores allow for the entry of CO2 into the leaf and for the exit of photosynthetically produced O2. The inevitable loss of water vapor by transpiration also occurs mainly through the stomatal pores, as we will discuss in Chapter 8 (Section 8.1B). Stomata thus serve as a control, helping to strike a balance between freely admitting the CO2 needed for photosynthesis and at the same time preventing excessive loss of water vapor from the plant. Air pollutants such as ozone (O2), nitrous oxide (NO), and sulfur dioxide (SO2) also enter plants primarily through the open stomata.
1.1C. Vascular Tissue
The xylem and the phloem make up the vascular systems found contiguously in the roots, stems (Fig. 1-3), and leaves of plants. In a tree trunk the phloem constitutes a layer of the bark and the xylem constitutes almost all of the wood. The xylem provides structural support for land plants. Water conduction in the xylem of a tree often occurs only in the outermost annual ring, which lies just inside the vascular cambium (region of meristematic activity from which xylem and phloem cells differentiate). Outside the functioning phloem are other phloem cells that can be shed as pieces of bark slough off. Phloem external to the xylem, as in a tree, is the general pattern for the stems of plants. As we follow the vascular tissue from the stem along a petiole and into a leaf, we observe that the xylem and the phloem often form a vein, which sometimes conspicuously protrudes from the lower surface of a leaf. Reflecting the orientation in the stem or the trunk, the phloem is found abaxial to the xylem in the vascular tissue of a leaf (i.e., the phloem is located on the side of the lower epidermis). The vascular system branches and rebranches as it crosses a dicotyledonous leaf, becoming smaller (in cross section) at each step. In contrast to the reticulate venation in dicotyledons, monocotyledons characteristically have parallel-veined leaves. Individual mesophyll cells in the leaf are never further than a few cells from the vascular tissue.
The movement of water and nutrients from the soil to the upper portions of a plant occurs primarily in the xylem. The xylem sap usually contains about 10 mol m-3(10 mM) inorganic nutrients plus organic forms of nitrogen that are metabolically produced in the root. The xylem is a tissue of various cell types that we will consider in more detail in the final chapter (Section 9.4B,D), when water movement in plants is discussed quantitatively. The conducting cells in the xylem are the narrow, elongated tracheids and the vessel members (also called vessel elements), which tend to be shorter and wider than the tracheids. Vessel members are joined end-to-end in long linear files; their adjoining end walls or perforation plates have from one large hole to many small holes. The conducting cells lose their protoplasts, and the remaining cell walls thus form a low-resistance channel for the passage of solutions. Xylem sap moves from the root, up the stem, through the petiole, and then to the leaves in these hollow dead xylem "cells," with motion occurring in the direction of decreasing hydrostatic pressure. Some solutes leave the xylem along the stem on the way to a leaf, and others diffuse or are actively transported across the plasma membranes of various leaf cells adjacent to the conducting cells of the xylem.
(Continues...)
Excerpted from Physicochemical and Environmental by Park S. Nobel Copyright © 2009 by Elsevier Inc.. Excerpted by permission of Academic Press. All rights reserved. No part of this excerpt may be reproduced or reprinted without permission in writing from the publisher.
Excerpts are provided by Dial-A-Book Inc. solely for the personal use of visitors to this web site.
Table of Contents
Cells and DiffusionWater
Solutes
Light
Photochemistry of Photosynthesis
Bioenergetics
Temperature and Energy Budgets
Leaves and Fluxes
Plants&Fluxes
Problem Solutions
Appendices
What People are Saying About This
The gold standard of plant science