Process Intensification: Design Methodologies / Edition 1 available in Paperback
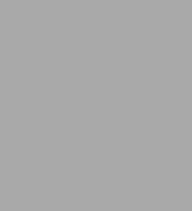
Process Intensification: Design Methodologies / Edition 1
- ISBN-10:
- 3110596075
- ISBN-13:
- 9783110596076
- Pub. Date:
- 10/21/2019
- Publisher:
- De Gruyter
- ISBN-10:
- 3110596075
- ISBN-13:
- 9783110596076
- Pub. Date:
- 10/21/2019
- Publisher:
- De Gruyter
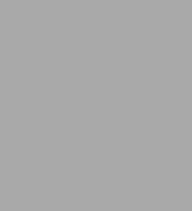
Process Intensification: Design Methodologies / Edition 1
Paperback
Buy New
$108.99-
PICK UP IN STORE
Your local store may have stock of this item.
Available within 2 business hours
Overview
Product Details
ISBN-13: | 9783110596076 |
---|---|
Publisher: | De Gruyter |
Publication date: | 10/21/2019 |
Series: | De Gruyter STEM |
Pages: | 338 |
Product dimensions: | 6.69(w) x 9.45(h) x (d) |
Age Range: | 18 Years |
About the Author
Table of Contents
List of contributors xiii
1 Generalities about process intensification Nelly Ramírez Corona Adriana Palacios Rosas 1
1.1 Introduction 1
1.2 PI principles 2
1.3 Devices and equipment in PI 3
1.4 PI methodologies 4
1.5 PI safety and control properties 6
1.6 Sustainability 7
1.7 Main drawbacks for PI implementation 8
1.8 PI assessment examples 9
1.9 Final remarks 13
References 13
2 Microreactors: Design methodologies, technology evolution, and applications to biofuels production Efraín Quiroz-Pérez Julio Armando de Lira-Flores Claudia Gutiérrez-Antonio 15
2.1 Introduction 15
2.2 Design methodologies 17
2.2.1 Design based on the analysis of parameters of microreactors performance 18
2.2.1.1 Mixing index 19
2.2.1.2 Mean variables 19
2.2.1.3 Figure of merit 20
2.2.1.4 Nonuniformity of flow 20
2.2.1.5 Studies based on the analysis of parameters related to microreactor performance 21
2.2.2 Design based on optimization techniques 25
2.2.3 CFD-based studies applied to the design of microreactors for biofuels production 27
2.3 Applications of microreactors to biofuels production 29
2.3.1 Liquid biofuels 29
2.3.1.1 Biomethanol 29
2.3.1.2 Biodiesel 30
2.3.1.3 Other processes 31
2.3.2 Gaseous biofuels 32
2.3.2.1 Renewable hydrogen 32
2.3.2.2 Synthetic gas natural 33
2.3.2.3 Synthesis gas 33
2.4 Technological advances for biofuels production 34
2.5 Recapitulation 43
References 45
3 Heat transfer enhancement technologies for improving heat exchanger performance Martín Picón-Núñez Jorge Luis García-Castillo Jorge C. Melo-González 51
3.1 Introduction 51
3.2 Thermal and hydraulic principles 53
3.3 Extended surfaces 55
3.3.1 Flat plate surfaces 55
3.3.2 Secondary surfaces 58
3.3.3 Extended surfaces for tubes 59
3.4 Turbulence promoters for tubular geometries 63
3.4.1 Benefits of the use of turbulence promoters. Illustrative example 1 64
3.4.2 Benefits of the use of turbulence promoters. Illustrative example 2 65
3.4.3 Thermo-hydraulic performance comparison of turbulence promoters 66
3.5 Heat transfer intensification in heat recovery networks 68
3.6 Conclusions 72
Nomenclature 74
Greek Letters 75
Subscripts 75
Superscripts 75
References 76
4 Reactive absorption of carbon dioxide: Modeling insights Massimiliano Errico Claudio Madeddu Roberto Baratti 79
4.1 Introduction 79
4.2 Reactive absorption applications 81
4.2.1 Nitric acid production 82
4.2.2 The SCOT process 82
4.2.3 Carbon dioxide capture and storage (CCS) 82
4.3 CO2 reactive absorption: Solvent selection, reaction scheme, and thermodynamic 83
4.3.1 Solvent selection 84
4.3.2 Reaction scheme 84
4.3.3 Definition of the thermodynamic model 86
4.4 Process modeling: Equilibrium stage vs rate-based model 87
4.4.1 The equilibrium stage model 88
4.4.2 The rate-based model 89
4.4.2.1 Interphase material transfer 90
4.4.2.2 Modeling the bulk 91
4.4.2.3 Modeling the film 92
4.5 Rate-based parameters evaluation 94
4.5.1 Wetted surface area 94
4.5.2 Material transfer and heat transfer coefficients 95
4.5.3 Fractional liquid hold-up 95
4.6 Fluid dynamics analysis 96
4.6.1 Axial diffusion/dispersion: Peclet number definition 96
4.6.2 Back-mixing due to the countercurrent effect 98
4.6.3 The analysis of the number of segments 98
4.7 Model validation 100
4.7.1 Laboratory-scale pilot-plant 100
4.7.2 Large-scale pilot-plant 102
4.7.3 Peclet number analysis 102
4.7.4 Component definition 105
4.7.5 Run T20: rate-based mode set-up 107
4.7.5.1 Analysis of the number of segments 108
4.7.5.2 Kinetic parameters calibration 112
4.7.5.3 Influence of the different flow models 113
4.7.6 Run 1-A2: extension to the large-scale plant 114
4.8 Conclusion 115
Bibliography 116
Notation 122
5 Optimal design methodology for homogeneous azeotropic distillation columns Abel Briones-Ramírez Claudia Gutiérrez-Antonio 125
5.1 Introduction 125
5.2 Optimal design methodology 130
5.2.1 Calculation of number of stages 130
5.2.2 Calculation of the minimum reflux ratio 132
5.2.3 Location of the feed stage 133
5.3 Cases of study 135
5.4 Results 136
5.4.1 Mixture acetone-isopropanol-water 136
5.4.2 Mixture acetone-chloroform-benzene 139
5.4.3 Mixture ethanol-water-ethylene glycol 140
5.4.4 Mixture methanol-isopropanol-water 141
5.5 Conclusions 142
Exercises 142
References 143
6 Graphical Tools for Designing Intensified Distillation Processes: Methods and Applications Nima Nazemzadeh Isuru A. Udugama Rasmus Fjordbak Nielsen Kristian Meyer Eduardo S. Perez-Cisneros Mauricio Sales-Cruz Jakob Kjøbsted Huusom Jens Abildskov Seyed Soheil Mansouri 145
6.1 Introduction 145
6.2 Shortcut methods for distillation column design 149
6.3 Why graphical methods as shortcut design tools for distillation processes? 151
6.3.1 Driving force approach 154
6.3.2 McCabe-Thiele method 155
6.3.3 Ponchon-Savarit method 155
6.4 Intensified distillation columns: Graphical design 156
6.4.1 Heat Integrated Distillation 156
6.4.1.1 Graphical design methods 156
6.4.1.1.1 McCabe-Thiele method for HIDiC 156
6.4.1.1.2 Ponchon-Savarit method for HIDiC 158
6.4.1.2 Graphical design of HIDiC 161
6.4.1.2.1 HIDiC design: McCabe-Thiele application 162
6.4.1.2.2 HIDiC design: Ponchon-Savarit application 163
6.4.2 Cyclic distillation design 163
6.4.2.1 Method 164
6.4.2.2 Case study 165
6.4.3 Reactive Distillation Design: Driving Force Approach 167
6.4.3.1 Method 167
6.4.3.2 Case study 168
6.5 Discussion and perspective 171
6.6 Concluding remarks 173
References 174
Appendix 177
7 Optimization Methodologies for Intensified Distillation Processes with Flexible Heat Integration Networks J. Rafael Alcántara-Avila 181
7.1 Introduction 181
7.2 Structures with heat integration 182
7.2.1 Heat integration 183
7.3 Optimization of heat-integrated structures 184
7.3.1 Mathematical formulation 187
7.3.2 Optimization methodology 196
7.3.3 Iterative optimization procedure 198
7.4 Case study 200
7.5 Results and discussion 202
7.5.1 Binary mixture separation 202
7.5.2 Multicomponent mixture separation 205
7.6 Conclusions 208
References 209
8 Conception, Design, and Development of Intensified Hybrid-Bioprocesses Oscar Andrés Prado-Rubio Javier Fontalvo John M. Woodley 211
8.1 Introduction 212
8.2 Overview of hybrid technologies for bioprocesses intensification 213
8.3 Methodologies for hybrid bioprocesses design 216
8.4 Hybrid bioprocesses design - examples 219
8.4.1 Hybrid fermentation and pervaporation system 220
8.4.1.1 Mathematical model 221
8.4.1.2 Conventional fed-batch fermenter analysis 223
8.4.1.3 Hybrid system - Effect of when the units are integrated 224
8.4.1.4 Hybrid system - Effect of membrane selectivity 225
8.4.2 Hybrid fermentation and Donnan Dialysis 227
8.4.2.1 System description 227
8.4.2.2 System modeling 229
8.4.2.3 Results and discussions 232
8.5 Future perspectives 238
Nomenclature 239
Subscripts 239
Bibliography 239
9 Design of Hybrid Distillation and Vapor Permeation or Pervaporation Systems Juan Álvaro León Oscar Andrés Prado-Rubio Javier Fontalvo 243
9.1 Introduction 243
9.2 Basic configurations of hybrid distillation and membrane systems 244
9.3 Basic approaches for designing hybrid distillation and membrane systems 247
9.4 Pervaporation and vapor permeation 248
9.5 Membrane residue curve maps 249
9.6 Case studies 252
9.6.1 Sequential membrane and distillation units 252
9.6.2 Hybrid distillation-pervaporation system using pervaporation as a final distillate separation step 253
9.6.3 Hybrid distillation and membrane system for reducing the number of stages 257
9.6.4 Pervaporation or vapor permeation for multicomponent distillation 261
9.6.5 Hybrid distillation and pervaporation systems in a single column 264
Case study: acetone-isopropanol-water mixture 270
9.7 Nomenclature 272
Symbols 273
Subscript 273
9.8 Exercises 273
References 274
10 Lignocellulosic biofuels process synthesis and intensification: Superstructure-based methodology Paola Ibarra-Gonzalez Ben-Guang Rong 277
10.1 Introduction 278
10.1.1 Worldwide transportation fuels consumption 278
10.1.2 Biofuels projection 280
10.2 Lignocellulosic BtL transportation fuels production processes 282
10.2.1 Lignocellulosic biomass as source for BtL fuels 282
10.2.2 Thermochemical conversion of lignocellulosic biomass 283
10.2.3 Upgrading technologies to transportation fuels 284
10.3 Systematic methodology framework: Synthesis of lignocellulosic BtL process routes 284
10.4 Synthesis, design, and evaluation of lignocellulosic BtL process routes 287
10.4.1 Synthesis of lignocellulosic BtL production process routes: Conceptual design 287
10.4.1.1 Gasification- HTFT hydrocarbon production-FT hydrocarbon upgrading (PR-GHTUF) 287
10.4.1.2 Gasification- LTFT hydrocarbon production-FT hydrocarbon upgrading (PR-GLTUF) 289
10.4.1.3 Gasification- HTFT hydrocarbon production-FT hydrocarbon hydroprocessing (PR-GHTH) 290
10.4.1.4 Pyrolysis-Hydroprocessing (PR-PHS) 290
10.4.1.5 Pyrolysis-Catalytic Cracking (PR-PCC) 291
10.4.2 BtL process routes: Design and evaluation of process flowsheets 291
10.4.2.1 Computational Studies: Aspen Analysis 292
10.4.2.2 Flowsheets setup 292
10.4.2.3 BtL process routes: process flowsheets simulation and evaluation 295
10.5 Integrated superstructure synthesis 296
10.5.1 Superstructure formulation: Processing blocks definition 296
10.5.2 Detailed superstructure to simplified superstructure: Lumped process blocks definition 297
10.5.3 Mass and energy integration 302
10.5.3.1 Mass integration 302
10.5.3.2 Energy integration 303
10.6 Mathematical modeling: Superstructure setting and MINLP solution 304
10.6.1 Superstructure setting 304
10.6.2 MINLP solution: Global optimal scenarios 308
10.7 Optimal scenarios evaluation with process simulator 309
10.8 Upgrading section configuration alternatives and possible intensifications 316
10.9 Conclusions 320
10.10 Nomenclature 321
References 323
Index 327