Solar Technologies for Buildings / Edition 1 available in Hardcover
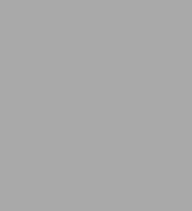
Solar Technologies for Buildings / Edition 1
- ISBN-10:
- 047148637X
- ISBN-13:
- 9780471486374
- Pub. Date:
- 07/18/2003
- Publisher:
- Wiley
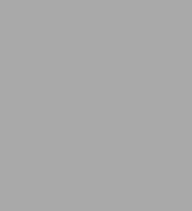
Solar Technologies for Buildings / Edition 1
Hardcover
Buy New
$225.95Overview
* Provides practical examples and calculations to enable component and system simulation e.g. Calculation of U-values, I-V curve parameters and radiance distribution modelling
* Discusses the new trends in thermal energy use, including the architectural integration of collector systems, integrated ventilation photovoltaics facades and solar powered absorption cooling systems
* Coverage of cutting-edge applications such as active and passive cooling techniques and results from ongoing research projects
Product Details
ISBN-13: | 9780471486374 |
---|---|
Publisher: | Wiley |
Publication date: | 07/18/2003 |
Pages: | 336 |
Product dimensions: | 6.85(w) x 9.70(h) x 0.95(d) |
About the Author
Read an Excerpt
Solar Technologies for Buildings
By Ursula Eicker
John Wiley & Sons
ISBN: 0-471-48637-XChapter One
1 Solar energy use in buildings1.1 Energy consumption of buildings
Buildings account today for about 40% of the final energy consumption of the European Union, with a large energy saving potential of 22% in the short term (up to 2010). Under the Kyoto protocol, the European Union has committed itself to reducing the emission of greenhouse gases by 8% in 2012 compared to the level in 1990, and buildings have to play a major role in achieving this goal. The European Directive for Energy Performance of buildings adopted in 2002 (to be implemented by 2005) is an attempt to unify the diverse national regulations, to define minimum common standards on buildings' energy performance and to provide certification and inspection rules for heating and cooling plants. While there are already extensive standards on limiting heating energy consumption (EN832 and prEN 13790), cooling requirements and daylighting of buildings are not yet set by any European standard. The reduction of energy consumption in buildings is of high socio-economic relevance, with the construction sector as Europe's largest industrial employer representing an annual investment of 868 x [10.sup.9] [euro] (2001) corresponding to 10% of gross domestic product. Almost two million companies, 97% of them small and medium enterprises, employ more than 8 million people (European Commission, 1997).
The distribution ofenergy use varies with climatic conditions. In Germany, where 44% of primary energy is consumed in buildings, 32% is needed for space heating, 5% for water heating, 2% for lighting and about 5% for other electricity consumption in residential buildings (Diekmann, 1997). The dominance of heat-consumption, almost 80% of the primary energy consumption of households, is caused by low thermal insulation standards in existing buildings, in which today 90% and even in 2050 60% of residential space will be located (Ministry for Transport and Buildings, Germany, 2000).
Since the 1970s oil crisis the heating energy requirement, particularly of new buildings, has been continuously reduced by gradually intensified energy legislation. With high heat insulation standards and the ventilation concept of passive houses, a low limit of heat consumption has meanwhile been achieved, which is around 20 times lower than today's values. A crucial factor for low consumption of passive buildings is the development of new glazing and window technologies, which enable the window to be a passive solar element and at the same time cause only low transmission heat losses. In new buildings with low heating requirements other energy consumption in the form of electricity for lighting, power and air conditioning, as well as in the form of warm water in residential buildings, is becoming more and more dominant. Electricity consumption within the European Union is estimated to rise by 50% by 2020. In this area renewable sources of energy can make an important contribution to the supply of electricity and heat.
1.1.1 Residential buildings
Due to the wide geographical extent of the European Union of nearly 35° geographical latitude difference (36° in Greece, 70° in northern Scandinavia), a wide range of climatic boundary conditions are covered. In Helsinki (60.3° northern latitude), average exterior air temperatures reach -6°C in January, when southern cities such as Athens at 40° latitude still have averages of +10°C. Consequently the building standards vary widely: whereas average heat transfer coefficients (U-values) for detached houses are 1 W/[m.sup.2]K in Italy, they are only 0.4 W/[m.sup.2]K in Finland. The heating energy demand determined using the European standard EN 832 is comparable in both cases at about 50 kWh/[m.sup.2]a.
If existing building standards are improved to the so-called passive building standard, heating energy consumption can be lowered to less than 20 kWh/[m.sup.2]a. The required U-values for the building shell are listed below for both current practice buildings and passive buildings.
The resulting heating energy requirement for current building practice varies between 55 kWh/[m.sup.2]a in Stockholm/Sweden and 93 kWh/[m.sup.2]a in Helsinki/Finland. These values can be lowered by nearly 80% when applying better insulation to the external surfaces and reducing ventilation losses.
Independent of the standard of insulation, water heating is always necessary in residential buildings, and this lies between about 220 (low requirement) and 1750 kWh per person per year (high requirement), depending on the pattern of consumption. For the middle requirement range of 30-60 litres per person and day, with a warm-water temperature of 45°C, the result is an annual consumption of 440-880 kWh per person, i.e. 1760-3520 kWh for an average four-person household. Related to a square metre of heated residential space, an average value of 25 kWh/[m.sup.2]a is often taken as a base.
The average electricity consumption of private households, around 3600 kWh per household per year, is of a similar order of magnitude. Related to a square metre of heated residential space, an average value of 31 kWh/[m.sup.2]a is the result. An electricity-saving household needs only around 2000 kWh/a. In a passive building project in Darmstadt (Germany), consumptions of between 1400 and 2200 kWh per household per year were measured, which corresponds to an average value of 11.6 kWh/[m.sup.2]a. Low energy buildings today have heat requirements of between 30 and 70 kWh/[m.sup.2]a.
1.1.2 Office and administrative buildings
Existing office and administrative buildings have approximately the same consumption of heat as residential buildings and most have a higher electricity consumption. According to a survey of the energy consumption of public buildings in the state of Baden-Wuerttemberg in Germany the average consumption of heat is 217 kWh/[m.sup.2]a, with an average electricity consumption of 54 kWh/[m.sup.2]a. The specific energy consumption of naturally ventilated office buildings in Great Britain is in a similar range of 200-220 kWh/[m.sup.2]a for heating and 48-85 kWh/[m.sup.2]a for electricity consumption (Zimmermann, Andersson, 1998). If the final energy consumption for heat and electricity is converted to primary energy consumption, comparable orders of magnitude of both energy proportions result. Still more important are the slightly higher costs of electricity.
Both heat and electricity consumption depend strongly on the building's use. In terms of the specific costs, electricity almost always dominates.
If one compares the energy costs of commercial buildings with the remaining current monthly operating costs, the relevance of a cost-saving energy concept is also apparent here: more than half of the running costs are accounted for by energy and maintenance. A large part of the energy costs is due to ventilation and air conditioning.
Heat consumption in administrative buildings can be reduced without difficulty, by improved thermal insulation, to under 100 kWh/[m.sup.2]a, and even to a few kWh per square metres and year in a passive building. Related to average consumption in the stock, a reduction to 5-10% is possible. Electricity consumption dominates total energy consumption where the building shell is energy-optimised and can be reduced by 50% at most. Even in an optimised passive energy office building in southern Germany, the electricity consumption remained at about 33 kWh/[m.sup.2]a, mainly due to the consumption of energy by office equipment such as computers.
While the measured values for heat consumption correspond well with the planned values, the measured total electricity consumption exceeds the planned value of 23.5 kWh/[m.sup.2]a by 42%.
A survey of good practice in office buildings in Britain showed that the electricity consumption in naturally ventilated offices is 36 kWh/[m.sup.2]a for a cellular office type, rising to 61 kWh/[m.sup.2]a for an open plan office and up to 132 kWh/[m.sup.2]a for an air-conditioned office (Zimmermann, 1999).
1.1.3 Air conditioning
In Europe energy consumption for air conditioning is rising rapidly. This is due to increased internal loads through electrical office appliances, but also to increased demand for comfort in summer. Summer overheating in highly glazed buildings is often an issue in modern office buildings, even in northern European climates. This unwanted and often unforeseen summer overheating leads to the curious fact that air conditioned buildings in northern Europe sometimes consume more cooling energy than those in Southern Europe that have a more obvious architectural emphasis on summer comfort. According to an analysis of a range of office buildings, an average of 40 kWh/[m.sup.2]a was obtained for southern climates, whereas 65 kWh/[m.sup.2]a were measured in northern European building projects (Mat Santamouris, University of Athens, private communication, 2002).
The largest European air conditioning manufacturer and consumer is Italy, accounting for nearly half of all European production (Adnot, 1999). Sixty-nine per cent of all room air conditioner sales are split units, with total annual sales of about 2 million units. In 1996 the total number of air conditioning units installed in Europe was about 7 500 000 units. Between 1990 and 1996 the electricity consumption for air conditioning in the European Union has risen from about 1400 GWh/year to 11 000 GWh/year and further increases up to 28 000 GWh/year are predicted by Adnot for the year 2010. Without any policy intervention or technological change for solar or waste heat-driven cooling machines, the associated C[O.sub.2] emissions will rise from 0.6 million tons in 1990 to 12 million tons in 2010. The average coefficients of performance for all cooling technologies is currently about 2.7 (cooling power to electricity input), with a target of about 3.0 for 2015.
Cooling energy is often required in commercial buildings, with the highest consumption world-wide in the USA. In Europe the cooling energy demand for such buildings varies between 3 and 30 MWh/year. Very little data is available for area-related cooling energy demand. Breembroek and Lazaro (1999) quote values between 20 kWh/[m.sup.2]a for Sweden, 40-50 kWh/[m.sup.2]a for China and 61 kWh/[m.sup.2]a for Canada.
Under German climatic conditions, demand for air conditioning exists only in administrative buildings with high internal loads, provided of course that external loads transmitted via windows are reduced effectively by sun-protection devices. In such buildings, the average summer electricity consumption for the operation of compression refrigerant plants is about 50 kWh/[m.sup.2]a, i.e. the primary energy requirement for airconditioning is 150 kWh/[m.sup.2]a, higher than the heating energy consumption of new buildings (Franzke, 1995).
In Southern Europe, the installed cooling capacity is often dominated by the residential market. Although in Spain less than 10% of homes have air conditioning systems, 71% of the installed cooling capacity is in the residential sector (Granados, 1997).
About 50% of internal loads are caused by office equipment such as PC's (typically 150 W including the monitor), printers (190 W for laser printers, 20 W for inkjets), photocopiers (1100 W) etc., which leads to an area-related load of about 10-15 W/[m.sup.2]. Modern office lighting has a typical connected load of 10-20 W/[m.sup.2] at an illuminance of 300-500 1x. The heat given off by people, around 5 W/[m.sup.2] in an enclosed office or 7 W/[m.sup.2] in an open-plan one, is also not negligible. Typical mid-range internal loads are around 30 W/[m.sup.2] or a daily cooling energy of 200 Wh/[m.sup.d], in the high range between 40-50 W/[m.sup.2] and 300 Wh/[m.sup.2]d (Zimmermann, 1999).
External loads depend greatly on the surface proportion of the glazing as well as the sun-protection concept. On a south-facing facade, a maximum irradiation of 600 W/[m.sup.2] occurs on a sunny summer day. The best external sun-protection reduces this irradiation by 80%. Together with the total energy transmission factor (g-value) of sun-protection glazing of typically 0.65, the transmitted external loads are about 78 W per square metre of glazing surface. In the case of a 3 [m.sup.2] glazing surface of an enclosed office, the result is a load of 234 W, which creates an external load of just about 20 W/[m.sup.2] based on an average surface of 12 [m.sup.2]. This situation is illustrated in the Figure 1.9 for south, east and west-facing facades in the summer:
The reducing coefficients of sun-protection devices depend particularly on the arrangement of the sun protection: external sun protection can reduce the energy transmission of solar radiation by 80%, whereas with sun protection on the inside a reduction of at most 60% is possible.
The total external and internal loads leads to an average cooling load in administrative buildings of around 50 W/[m.sup.2].
1.2 Meeting requirements by active and passive solar energy use
1.2.1 Active solar energy use for electricity, heating and cooling
Active solar-energy use in buildings today contributes primarily to meeting electricity requirements by photovoltaics, and to warm water heating by solar thermal collectors. Meeting the space heating requirement by solar thermal systems is recommended if conventional heat insulation potential is fully exhausted or if special demands such as monument protection or facade retention do not permit external insulation. Support heating with thermal collectors, with small contributions of approximately 10-30%, is always possible without significant surface-specific losses. Outside-air pre-heating with thermal air collectors can also make a significant contribution to reducing ventilation heat losses.
In air-conditioned buildings, thermal cooling processes such as open and closed sorption processes can be powered by active solar components.
When considering the potential solar contribution to the different energy requirements in buildings (heating, cooling, electricity), it is necessary to analyse the solar irradiance, the transformation efficiency of the solar technology in question and the available surface potential in buildings as well as the economically usable potential.
For a first design of a solar energy system, it is usually sufficient to consider the annual solar energy supply on the receiver surface. The maximum annual irradiance is achieved in the northern hemisphere on south-facing surfaces inclined at an angle of the geographical latitude minus about 10°. In Stuttgart the maximum irradiation on a 38° inclined southfacing surface is 1200 kWh/[m.sup.2]a. A deviation from south orientation of + or - 50° leads to an annual irradiation reduction of 10%. A south-facing facade receives about 72% of the maximum possible irradiation [G.sub.max] (defined in Figure 1.12 as 100%).
Continues...
Excerpted from Solar Technologies for Buildings by Ursula Eicker Excerpted by permission.
All rights reserved. No part of this excerpt may be reproduced or reprinted without permission in writing from the publisher.
Excerpts are provided by Dial-A-Book Inc. solely for the personal use of visitors to this web site.
Table of Contents
Preface ixAbbreviations in the text xi
1 Solar energy use in buildings 1
1.1 Energy consumption of buildings 1
1.1.1 Residential buildings 2
1.1.2 Office and administrative buildings 4
1.1.3 Air conditioning 6
1.2 Meeting requirements by active and passive solar energy use 9
1.2.1 Active solar energy use for electricity, heating and cooling 9
1.2.2 Meeting heating energy requirements by passive solar energy use 12
2 Solar irradiance 13
2.1 Extraterrestrial solar irradiance 13
2.1.1 Power and spectral distribution of solar irradiance 13
2.1.2 Sun–Earth geometry 16
2.1.2.1 Equator coordinates 17
2.1.2.2 Horizon coordinates 20
2.1.2.3 Sun-position diagrams 22
2.2 The passage of rays through the atmosphere 24
2.3 Statistical production of hourly irradiance data records 26
2.3.1 Daily average values from monthly average values 27
2.3.2 Hourly average values from daily average values 31
2.4 Global irradiance and irradiance on inclined surfaces 34
2.4.1 Direct and diffuse irradiance 34
2.4.2 Conversion of global irradiance to inclined surfaces 35
2.4.2.1 An isotropic diffuse irradiance model 35
2.4.2.2 Diffuse irradiance model based on Perez 36
2.4.3 Measurement techniques for solar irradiance 39
2.5 Shading 39
3 Solar thermal energy 45
3.1 Solar-thermal water collectors 45
3.1.1 Innovations 45
3.1.2 System overview 46
3.1.3 Thermal collector types 47
3.1.3.1 Swimming pool absorbers 47
3.1.3.2 Flat plate collectors 47
3.1.3.3 Vacuum tube collectors 48
3.1.3.4 Parabolic concentrating collectors 48
3.1.4 System engineering for heating drinking-water 49
3.1.4.1 The solar circuit and hydraulics 49
3.1.4.2 Heat storage 55
3.1.4.3 Piping and circulation losses 60
3.1.5 System technology for heating support 61
3.1.6 Large solar plants for heating drinking water with short-term stores 63
3.1.6.1 Design of large solar plants 66
3.1.7 Solar district heating 68
3.1.8 Costs and economy 71
3.1.9 Operational experiences and relevant standards 73
3.1.10 Efficiency calculation of thermal collectors 74
3.1.10.1 Temperature distribution of the absorber 75
3.1.10.2 Collector efficiency factor F' 79
3.1.10.3 Heat dissipation factor FR 79
3.1.10.4 Heat losses of thermal collectors 83
3.1.10.5 Optical characteristics of transparent covers and absorber materials 92
3.1.11 Storage modelling 97
3.2 Solar air collectors 103
3.2.1 System engineering 105
3.2.2 Calculation of the available thermal power of solar air collectors 107
3.2.2.1 Temperature-dependent material properties of air 107
3.2.2.2 Energy balance and collector efficiency factor 108
3.2.2.3 Convective heat transfer in air collectors 109
3.2.2.4 Thermal efficiency of air collectors 117
3.2.3 Design of the air circuit 120
3.2.3.1 Collector pressure losses 120
3.2.3.2 Air duct systems 121
4 Solar cooling 123
4.1 Open cycle desiccant cooling 125
4.1.1 Introduction to the technology 125
4.1.2 Coupling with solar thermal collectors 128
4.1.3 Costs 128
4.1.4 Physical and technological bases of sorption-supported air-conditioning 129
4.1.4.1 Technology of sorption wheels 129
4.1.4.2 Air-status calculations 130
4.1.4.3 Dehumidifying potential of sorption materials 132
4.1.4.4 Calculation of the sorption isotherms and isosteres of silica gel 135
4.1.4.5 Calculation of the dehumidifying performance of a sorption rotor 140
4.1.5 The technology of heat recovery 143
4.1.5.1 Recuperators 143
4.1.5.2 Regenerative heat exchangers 148
4.1.6 Humidifier technology 152
4.1.7 Design limits and climatic boundary conditions 153
4.1.7.1 Demands on room temperatures and humidities 153
4.1.7.2 Regeneration temperature and humidity 153
4.1.7.3 Calculation of supply air status with different climatic boundary conditions 154
4.1.7.4 Limits and application possibilities of open sorption 155
4.1.8 Energy balance of sorption-supported air-conditioning 156
4.1.8.1 Usable cooling power of open sorption 156
4.1.8.2 Coefficients of performance and primary energy consumption 158
4.2 Closed cycle adsorption cooling. 162
4.2.1 Technology and areas of application 162
4.2.2 Costs 163
4.2.3 Operational principle 163
4.2.4 Energy balances and pressure conditions 165
4.2.4.1 Evaporator 166
4.2.4.2 Condenser 168
4.2.4.3 The adsorption process 169
4.2.4.4 Heating phase 172
4.2.4.5 The desorption process 172
4.2.4.6 Cooling phase 174
4.2.5 Coefficients of performance 175
4.3 Absorption cooling technology 177
4.3.1 The absorption cooling process and its components 178
4.3.1.1 Double-lift absorption cooling process 181
4.3.1.2 Evaporator and condenser 182
4.3.1.3 Absorber 183
4.3.1.4 Generator 185
4.3.2 Physical principles of the absorption process 185
4.3.2.1 Vapour pressure curves of material pairs 185
4.3.3 Refrigerant vapour concentration 189
4.3.4 Energy balances and performance figures of an absorption cooler 190
4.3.4.1 Ideal performance figures 190
4.3.4.2 Real performance figures and enthalpy balances 191
4.3.5 Absorption technology and solar plants 200
5 Grid-connected photovoltaic systems 201
5.1 Structure of grid-connected systems 201
5.2 Solar cell technologies 203
5.3 Module technology 203
5.4 Building integration and costs 204
5.5 Energy production and the performance ratio of PV systems 205
5.5.1 Energy amortisation times 206
5.6 Physical fundamentals of solar electricity production 207
5.7 Current-voltage characteristics 209
5.7.1 Characteristic values and efficiency 209
5.7.2 Curve fittings to the current-voltage characteristic 210
5.7.2.1 Parameter adjustment from module data sheets 216
5.7.2.2 Full parameter set calculation 220
5.7.2.3 Simple explicit model for system design 221
5.7.3 I-V characteristic addition and generator interconnecting 223
5.8 PV performance with shading. 225
5.8.1 Bypass diodes and backwards characteristics of solar cells 225
5.9 Simple temperature model for PV modules 228
5.10 System engineering 231
5.10.1 DC connecting 231
5.10.1.1 Cable sizing 231
5.10.1.2 System voltage and electrical safety 232
5.10.1.3 String diodes and short-circuit protection 232
5.10.2 Inverters 234
5.10.2.1 Operational principle 234
5.10.2.2 Electrical safety and mains monitoring 235
5.10.2.3 Inverter efficiencies 235
5.10.2.4 Power sizing of inverters 238
6 Thermal analysis of building-integrated solar components 243
6.1 Empirical thermal model of building-integrated photovoltaics 244
6.2 Energy balance and stationary thermal model of ventilated double facades 246
6.2.1 Heat transfer coefficients for the interior and facade air gap 250
6.3 Building-integrated solar components (U- and g-values) 254
6.4 Warm-air generation by photovoltaic facades 257
7 Passive solar energy 260
7.1 Passive solar use by glazings 260
7.1.1 Total energy transmittance of glazings 261
7.1.2 Heat transfer coefficients of windows 263
7.1.3 New glazing systems 265
7.2 Transparent thermal insulation 265
7.2.1 Operational Principle 266
7.2.2 Materials used and construction 270
7.2.2.1 Construction principles of TWD systems 270
7.3 Heat storage by interior building elements 271
7.3.1 Component temperatures for sudden temperature increases 274
7.3.2 Periodically variable temperatures 281
7.3.3 Influence of solar irradiance 286
8 Lighting technology and daylight use 288
8.1 Introduction to lighting and daylighting technology 288
8.1.1 Daylighting of interior spaces 289
8.1.2 Luminance contrast and glare 291
8.2 Solar irradiance and light flux 291
8.2.1 Physiological–optical basics 292
8.2.2 Photometric radiation equivalent 292
8.2.3 Artificial light sources. 294
8.3 Luminance and illuminance 295
8.3.1 Luminance and adaptation of the eye 299
8.3.2 Distribution of the luminous intensity of artificial light sources 300
8.3.3 Units and definitions 303
8.4 Sky luminous intensity models 304
8.5 Light measurements 307
8.6 Daylight distribution in interior spaces 308
8.6.1 Calculation of daylight coefficients 311
References 316
Index 320
What People are Saying About This
"...balances the physics and engineering background of solar heating, cooling and building integrated photovoltaics with practical applications..." (Bulletin, Vol 94(24/25), 2003)