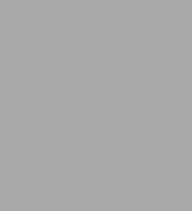
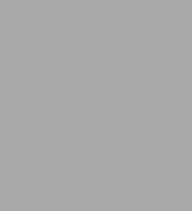
eBook
Available on Compatible NOOK devices, the free NOOK App and in My Digital Library.
Related collections and offers
Overview
The Chemistry of Polymers, 5th Edition, is fully updated with the latest developments in polymer science providing a highly readable textbook for those requiring a broad overview of the subject.
Like previous editions, the book continues to explore the subject from an applications point of view, providing a comprehensive introduction to all aspects of polymer science including synthesis, structure, properties, degradation and dendrimers. Recent advances in special topics in polymer chemistry and polymers and the environment are also discussed in an informative and up-to-date manner.
The new edition features additional content on recent developments in new polymer synthesis techniques including reversible addition-fragmentation chain transfer (RAFT) polymerization, atom transfer radical polymerization (ATRP) and ring-opening metathesis polymerization (ROMP). The book also contains new content on the latest developments in polymer characterisation methods as well as applications of polymers including co-ordination polymers and lithium-polymer batteries.
The book is essential reading for university students, teachers and scientists who wish to acquire an up-to-the-minute overview of polymer science and its many specialised topics in an informative and easy to read style.
Product Details
ISBN-13: | 9781788011143 |
---|---|
Publisher: | Royal Society of Chemistry |
Publication date: | 01/17/2023 |
Sold by: | Barnes & Noble |
Format: | eBook |
Pages: | 210 |
File size: | 2 MB |
About the Author
Read an Excerpt
The Chemistry of Polymers
By John W. Nicholson
The Royal Society of Chemistry
Copyright © 2017 John W. NicholsonAll rights reserved.
ISBN: 978-1-78801-114-3
CHAPTER 1
Polymer Chemistry
1.1 Basic Concepts
A polymer is a large molecule built up from numerous smaller molecules. These large molecules may be linear, slightly branched, or highly interconnected. In the latter case the structure develops into a large three-dimensional network.
The small molecules used as the basic building blocks for these large molecules are known as monomers. For example the commercially important material poly(vinyl chloride) is made from the monomer vinyl chloride. The repeat unit in the polymer usually corresponds to the monomer from which the polymer was made. There are exceptions to this, though. Poly(vinyl alcohol) is formally considered to be made up of vinyl alcohol (CHCHOH) repeat units but there is, in fact, no such monomer as vinyl alcohol. The appropriate molecular unit exists in the alternative tautomeric form, ethanal CHCHO. To make this polymer, it is necessary first to prepare poly(vinyl ethanoate) from the monomer vinyl ethanoate, and then to hydrolyse the product to yield the polymeric alcohol.
The size of a polymer molecule may be defined either by its mass (see Chapter 6) or by the number of repeat units in the molecule. This latter indicator of size is called the degree of polymerisation, DP. The relative molar mass of the polymer is thus the product of the relative molar mass of the repeat unit and the DP.
There is no clear cut boundary between polymer chemistry and the rest of chemistry. As a very rough guide, molecules of relative molar mass of at least 1000 or a DP of at least 100 are considered to fall into the domain of polymer chemistry.
The vast majority of polymers in commercial use are organic in nature, that is they are based on covalent compounds of carbon. This is also true of the silicones which, though based on silicon-oxygen backbones, also generally contain significant proportions of hydrocarbon groups. The other elements involved in polymer chemistry most commonly include hydrogen, oxygen, chlorine, fluorine, phosphorus, and sulfur, i.e. those elements which are able to form covalent bonds, albeit of some polarity, with carbon.
As is characteristic of covalent compounds, in addition to primary valence forces, polymer molecules are also subject to various secondary intermolecular forces. These include dipole forces between oppositely charged ends of polar bonds and dispersion forces which arise due to perturbations of the electron clouds about individual atoms within the polymer molecule. Hydrogen bonding, which arises from the particularly intense dipoles associated with hydrogen atoms attached to electronegative elements such as oxygen or nitrogen, is important in certain polymers, notably proteins. Hydrogen bonds have the effect of fixing the molecule in a particular orientation. These fixed structures are essential for the specific functions that proteins have in the biochemical processes of life.
1.2 The History of the Concept of the Macromolecule
Modern books about polymer chemistry explain that the word polymer is derived from the Greek words 'poly' meaning many and 'meros' meaning part. They often then infer that it follows that this term applies to giant molecules built up of large numbers of interconnected monomer units. In fact this is misleading since historically the word polymer was coined for other reasons. The concept of polymerism was originally applied to the situation in which molecules had identical empirical formulae but very different chemical and physical properties. For example, benzene (CH; empirical formula CH) was considered to be a polymer of acetylene (CH; empirical formula also CH). Thus the word 'polymer' is to be found in textbooks of organic chemistry published up to about 1920 but not with its modern meaning.
The situation is confused, however, by the case of certain chemicals. Styrene, for example, was known from the mid-nineteenth century as a clear organic liquid of characteristic pungent odour. It was also known to convert itself under certain circumstances into a clear resinous solid that was almost odour-free, this resin then being called metastyrene. The formation of metastyrene from styrene was described as a polymerisation and metastyrene was held to be a polymer of styrene. However, these terms applied only in the sense that there was no change in empirical formula despite the very profound alteration in chemical and physical properties. There was no understanding of the cause of this change and certainly the chemists of the time had no idea of what had happened to the styrene that was remotely akin to the modern view of polymerisation.
Understanding of the fundamental nature of those materials now called polymers had to wait until the 1920s, when Herman Staudinger coined the word 'macromolecule' and thus clarified thinking. There was no ambiguity about this new term – it meant 'large molecule', again from the Greek, and these days is used almost interchangeably with the word polymer. Strictly speaking, though, the words are not synonymous. There is no reason in principle for a macromolecule to be composed of repeating structural units; in practice, however, they usually are. Staudinger's concept of macromolecules was not at all well received at first. His wife once recalled that he had 'encountered opposition in all his lectures'. Typical of this opposition was that of one distinguished organic chemist who declared that it was as if zoologists 'were told that somewhere in Africa an elephant was found who was 1500 feet long and 300 feet high'.
There were essentially three reasons for this opposition. Firstly, many macromolecular compounds in solution behave as colloids. Hence they were assumed to be identical with the then known inorganic colloids. This in turn implied that they were not macromolecular at all, but were actually composed of small molecules bound together by ill-defined secondary forces. Such thinking led the German chemist C. D. Harries to pursue the search for the 'rubber molecule' in the early years of the twentieth century. He used various mild degradations of natural rubber, which he believed would destroy the colloidal character of the material and yield its constituent molecules, which were assumed to be fairly small. He was, of course, unsuccessful.
The second reason for opposition to Staudinger's hypothesis was that it meant the loss of the concept of a single formula for a single compound. Macromolecules had to be written in the form (CH2CHX)n where n was a large number. Moreover, no means were available, or indeed are available, for discretely separating molecules where n=100 from those where n=101. Any such attempted fractionation always gives a distribution of values of n and, even if the mean value of a fraction is actually n=100, there are significant numbers of molecules of n=99, n=101, and so on. Now the concept of one compound, one formula, with one formula being capable of both physical (i.e. spatial) and chemical interpretation, had been developed slowly and at some cost, with many long, hard-fought battles. Organic chemists could not easily throw it out, particularly in view of the fact that it had been so conspicuously successful with much of the rest of organic chemistry.
The third reason for opposition lay in the nature of many of the polymeric materials then known. Not only were they apparently ill-characterised, but they were also frequently non-crystalline, existing as gums and resins. Just the sort of unpromising media, in fact, from which dextrous organic chemists had become used to extracting crystalline substances of well characterised physical and chemical properties. To accept such resins as inherently non-crystallisable and not capable of purification in the traditional sense of the word was too much for the self-esteem of many professional organic chemists.
Staudinger's original paper opposing the prevalent colloidal view of certain organic materials was published in 1920 and contained mainly negative evidence. Firstly, he showed that the organic substances retained their colloidal nature in all solvents in which they dissolve; by contrast, inorganic colloids lose their colloidal character when the solvent is changed. Secondly, contrary to what would have been expected, colloidal character was able to survive chemical modification of the original substance.
By about 1930 Staudinger and others had accumulated much evidence in favour of the macromolecular hypothesis. The final part in establishing the concept was carried out by Wallace Carothers of the Du Pont company in the USA. He began his work in 1929 and stated at the outset that the aim was to prepare polymers of definite structure through the use of established organic reactions. Though his personal life was tragic, Carothers was an excellent chemist who succeeded brilliantly in his aim. By the end of his work he had not only demonstrated the relationship between structure and properties for a number of polymers, but he had invented materials of tremendous commercial importance, including neoprene rubber and the nylons.
1.3 Classification of Polymers
There are a number of methods of classifying polymers. One is to adopt the approach of using their response to thermal treatment and to divide them into thermoplastics and thermosets. Thermoplastics are polymers which melt when heated and resolidify when cooled, while thermosets are those which do not melt when heated but, at sufficiently high temperatures, decompose irreversibly. This system has the benefit that there is a useful chemical distinction between the two groups. Thermoplastics comprise essentially linear or lightly branched polymer molecules, while thermosets are substantially crosslinked materials, consisting of an extensive three-dimensional network of covalent chemical bonding.
Another classification system, first suggested by Carothers in 1929, is based on the nature of the chemical reactions employed in the polymerisation. Here the two major groups are the condensation and the addition polymers. Condensation polymers are those prepared from monomers where reaction is accompanied by the loss of a small molecule, usually of water, for example polyesters which are formed by the condensation shown in Reaction (1.1).
[MATHEMATICAL EXPRESSION OMITTED] (1.1)
By contrast, addition polymers are those formed by the addition reaction of an unsaturated monomer, such as takes place in the polymerisation of vinyl chloride (Reaction (1.2)).
nCH2 = CHCl [right arrow] [— CH2-CHCl–]n (1.2)
This system was slightly modified by P. J. Flory, who placed the emphasis on the mechanisms of the polymerisation reactions. He reclassified polymerisations as step reactions or chain reactions corresponding approximately to condensation or addition in Carother's scheme, but not completely. A notable exception occurs with the synthesis of polyurethanes, which are formed by reaction of isocyanates with hydroxy compounds and follow 'step' kinetics, but without the elimination of a small molecule from the respective units (Reaction (1.3)).
[MATHEMATICAL EXPRESSION OMITTED] (1.3)
In the first of these, the kinetics are such that there is a gradual build up of high relative molar mass material as reaction proceeds, with the highest molar mass molecules not appearing until the very end of the reaction. On the other hand, chain reactions, which occur only at a relatively few activated sites within the reacting medium, occur with rapid build up of a few high relative molar mass molecules while the rest of the monomer remains unreacted. When formed, such macromolecules stay essentially unchanged while the rest of the monomer undergoes conversion. This means that large molecules appear very early in the polymerisation reaction, which is characterised by having both high relative molar mass and monomer molecules present for most of the duration of the reaction.
Step reactions can give molecules having a variety of morphologies from the simple unbranched linear to the heavily crosslinked network. The final structure depends on the number of functional groups in the parent monomers – the greater the proportion with a functionality of greater than two, the more extensive will be the branching until, at sufficient degrees of branching, a highly crosslinked network emerges. Chain reactions, by contrast, give only linear or lightly branched polymers. Thus, in terms of the thermoplastic/thermoset classification, chain reactions give thermoplastics, while step reactions may give either thermoplastics or thermosets.
1.4 Structure and Properties of Polymers
Having established the basic principles of classification in polymer chemistry, we will now turn our attention to individual polymers and consider a little about their physical and chemical properties. Most of the examples which follow are of commercial importance, though it is their properties that are emphasised rather than the complete chemistry of their industrial manufacture. In terms of the classification systems just referred to, it is found that the polymers of major commercial importance tend to be the thermoplastics or addition polymers rather than the thermosets, and these will be dealt with first.
1.4.1 Poly(ethylene)
This polymer has one of the simplest molecular structures ([CH2CH2 –]n) and is at p resent the largest tonnage plastic material, having first been produced commercially in 1939 for use in electrical insulation. There is a difficulty over the nomenclature of this polymer. The IUPAC recommended name for the monomer is ethene, rather than the olderethylene. Hence the IUPAC name for the polymer is poly (ethene). However, this name is almost never used by chemists working with the material; throughout this book, therefore, this polymer will be referred to by its more widespread name, poly(ethylene).
There are four different industrial routes to the preparation of poly (ethylene), and they yield products having slightly different properties. The four routes are:
(i) High Pressure Processes,
(ii) Ziegler Processes,
(iii) The Phillips Process,
(iv) The Standard Oil (Indiana) Process.
The first group of these use pressures of 1000–3000 atm and temperatures of 80–300 °C. Free-radical initiators, such as benzoyl peroxide or oxygen, are generally used (see Chapter 2), and conditions need to be carefully controlled to prevent a runaway reaction, which would generate hydrogen, methane, and graphite rather than polymer. In general, high-pressure processes tend to yield lower density poly(ethylenes), typically in the range 0.915–0.945 g cm-3, which also have relatively low molar masses.
Ziegler processes are based on co-ordination reactions catalysed by metal alkyl systems. Such reactions were discovered by Karl Ziegler in Germany and developed by G. Natta at Milan in the early 1950s.
A typical Ziegler–Natta catalyst is the complex prepared from titanium tetrachloride and triethylaluminium. It is fed into the reaction vessel first, after which ethylene is added. Reaction is carried out at low pressures and low temperatures, typically no more than 70 °C, with rigorous exclusion of air and moisture, which would destroy the catalyst. The poly(ethylenes) produced by such processes are of intermediate density, giving values of about 0.945 g cm-3. A range of relative molar masses may be obtained for such polymers by varying the ratio of the catalyst components or by introducing a small amount of hydrogen into the reaction vessel.
Lastly the Phillips and the Standard Oil (Indiana) Processes both yield high density poly(ethylenes), using relatively low pressures and temperatures. Details of these processes are given in Table 1.1.
The polymer is a very familiar material in the modern world. It is a waxy solid which is relatively low in cost, easily processed, and shows good chemical resistance. Low relative molar mass grades have the disadvantage that they suffer from so-called 'environmental stress cracking', that is they suddenly fail catastro-phically for no apparent reason after exposure to sunlight and moisture. Despite this drawback, the various grades of poly(ethylene) have a wide range of uses. These include pipes, packaging, components for chemical plant, crates, and items for electrical insulation.
1.4.2 Poly(propylene)
This polymer, which has the structure [–CH2CH(CH3)–]n arose as a commercial material following the work of Natta on catalysts for the preparation of high relative molar mass polymers from alkenes. Following his work on the polymerisation of ethylene, Natta showed in 1954 that it was possible to prepare analogous polymers of propylene. Commercial exploitation followed rapidly, and poly (propylene) was first marketed in 1957.
Like poly(ethylene), there are formal problems with the nomenclature of this polymer, since its IUPAC name, poly(propene), is also rarely if ever used by polymer chemists. Since, in practice, no ambiguity is associated with the non-systematic name, this is the one that is generally used, as it will be throughout this book.
(Continues...)
Excerpted from The Chemistry of Polymers by John W. Nicholson. Copyright © 2017 John W. Nicholson. Excerpted by permission of The Royal Society of Chemistry.
All rights reserved. No part of this excerpt may be reproduced or reprinted without permission in writing from the publisher.
Excerpts are provided by Dial-A-Book Inc. solely for the personal use of visitors to this web site.