The Influence of Cooperative Bacteria on Animal Host Biology available in Hardcover
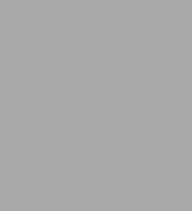
The Influence of Cooperative Bacteria on Animal Host Biology
- ISBN-10:
- 0521834651
- ISBN-13:
- 9780521834650
- Pub. Date:
- 08/22/2005
- Publisher:
- Cambridge University Press
- ISBN-10:
- 0521834651
- ISBN-13:
- 9780521834650
- Pub. Date:
- 08/22/2005
- Publisher:
- Cambridge University Press
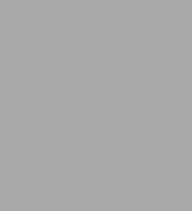
The Influence of Cooperative Bacteria on Animal Host Biology
Hardcover
Buy New
$156.00Overview
Product Details
ISBN-13: | 9780521834650 |
---|---|
Publisher: | Cambridge University Press |
Publication date: | 08/22/2005 |
Series: | Advances in Molecular and Cellular Microbiology , #10 |
Pages: | 454 |
Product dimensions: | 5.98(w) x 9.02(h) x 0.98(d) |
About the Author
Brian Henderson is Professor of Cell Biology and head of the Cellular Microbiology Research Group in the Division of Microbial Diseases, Eastman Dental Institute, University College London. He is the co-editor of Molecular Chaperones and Cell Signalling (2005), Bacterial Evasion of Host Immune Responses (2003), and Bacterial Disease Mechanisms (2002).
Edward G. Ruby is Professor of Medical Microbiology and Immunology at the University of Wisconsin School of Medicine.
Read an Excerpt
Cambridge University Press
0521834651 - The Influence of Cooperative Bacteria on Animal Host Biology - Role in the Interference with Cell Growth Regulation - Edited by Margaret J. McFall-Ngai, Brian Henderson and Edward G. Ruby
Excerpt
I Evolutionary biology of animal host-bacteria interactions
CHAPTER 1
How have bacteria contributed to the evolution of multicellular animals?
Carole S. Hickman
1.1. INTRODUCTION
Mutualistic interactions between animals and microbes have a long evolutionary history and a profound influence on the continuously changing shape of life on Earth. The impact of animal-microbial symbioses extends far beyond the ecological and evolutionary effects on the interacting partners to include fundamental modifications of the biosphere and geosphere. A new discipline uniting the divergent perspectives of zoology and microbiology on animal-microbe interactions must extend beyond the life sciences to include geology and the predominantly physical sciences of biogeochemistry and geomicrobiology. Astrobologists, exploring the properties of microbial consortia in extreme ecosystems (Cady 1998), and paleontologists, discovering new worlds of microbial complexity in ancient ecosystems (Hagadorn et al. 1999), are converging on principles of evolving partnerships and evidence of the same interconnectivity that are being sought by workers who envision a new field of beneficial animal-microbe interactions (Ruby et al. 2004).
This chapter establishes the geological context and examines the geologic and fossil evidence for three evolutionary propositions: (1) bacteria prepared the biosphere and played a major role in the origin of animals, (2) bacteria played a role in the subsequent diversification and shaping of animal life and its response to biotic crises, and (3) bacteria have influenced the evolution of animal development. The chapter also outlines a set of specific contributions that animal-microbe symbioses have made to the history and shape of life and presents a set of challenges for future research.
1.2. THE ROLE OF BACTERIA IN THE EVOLUTIONARY ORIGINS OF ANIMAL LIFE
1.2.1. A Perspective from Deep Time and Geology
The most striking fact of the fossil record of bacteria and animals is the strongly asymmetric timing of their origins (Fig. 1.1). Earth formed 4.5 billion years ago (Ga), and the first geochemical evidence (isotope ratios indicative of biological fractionation of carbon) for microbial activity appears in the rock record between 3.8 and 3.5 Ga (Mojzsis et al. 1996; Falkowski and Raven 1997; Rosing 1999). Prokaryotic fossils have been reported from rocks as old as 3.47 billion years (Schopf, 1993), and structural evidence of prokaryotic aggregations in the form of films, mats, and stromatolites dates to 3.5 Ga (Awramik 1984; Schopf 1992). Debates over the biogenicity of the very first putative cellular prokaryotes are peripheral to the central observation that prokaryotic life arose, diversified, and underwent more than 2 billion years of evolutionary change before the emergence of animals. Microbes have been the only form of life on Earth throughout most of its history. Animals first appear in the fossil record approximately 500 million years ago (Ma). By this time, microbes had profoundly modified the physical and chemical environments of the atmosphere, hydrosphere, and lithosphere, establishing the habitats in which multicellular life made its debut. Animals arose in a world teeming with microbes that had already established complex symbiotic interactions (both extra- and intracellular) with one another.
Figure 1.1. Major dated events and first appearances in the Precambrian geologic record. Evidence is based on fossils and sedimentary structures. Chemical and physical data are in the form of isotopes, biomarker molecules, biominerals, sedimentary structures, trace fossils, and body fossils. Hypothetical events, for which there is no physical or chemical evidence, are not included.
Image not available in HTML version |
Stromatolites and microbial mat textures are the most important features of Precambrian sedimentary rocks, providing evidence of the interaction of communities with the geosphere, both in terms of sediment trapping and binding, and in terms of biologically induced precipitation of calcium carbonate. Although modern stromatolite ecosystems contain some elements that were not present in the Precambrian, they provide models in both marine settings (Fig. 1.2a) and lakes (Fig. 1.2b) for the study of microbial interactions and collaborations. However, it is the 3.5 billion-year fossil record of stromatolites and microbial mats that holds the key to understanding the history and evolution of these miniature ecosystems as accumulations of successive symbiotic microbial structures.
1.2.2. A Paleo-environmental Perspective
Even though there is abundant and widespread fossil evidence of stromatolitic communities dominated by cyanobacteria by 3 Ga, it is puzzling that the first eukaryotic cells do not appear in the rock record for another billion years. The lag time in the emergence of eukaryotes is less perplexing in light of independent geologic evidence that a stable aerobic global environment was not established until approximately 2 Ga. What happened to the oxygen? The rock record offers a compelling suggestion of a major oxygen sink in the form of all the dissolved iron that accumulated in the oceans during early Earth history. The evidence that oxygen combined with soluble iron is manifest in the banded iron formations (BIFs), massive accumulations of iron oxides that formed during the early part of the Proterozoic. Study of the evolutionary roles of microbes in promoting mineral formation and in establishing control of geochemical transformations and geochemical cycles is in its infancy (Newman and Banfield 2002). Although microbial partnerships with the geosphere do not co-evolve in the biological sense of microbial partnerships with multicellular organisms, it may be useful to explore the relationships between these two kinds of interactions.
Figure 1.2. Living stromatolites provide diverse and complex examples of layered microbial ecosystems that can serve as a basis for study and modeling of microbial symbioses. a. Marine stromatolites in Shark Bay (Hamelin Pool), Western Australia. b. Non-marine stromatolites in Lake Clifton, Western Australia.
Image not available in HTML version |
Land and sea-surface temperatures during most of Earth history have been significantly warmer than they are today. Biogeochemical data as well as molecular genetic data suggest that prokaryotic life arose in the extreme environments of hydrothermal vents (Pace 1997). Thermally altered rocks provide the hard evidence that these are the most ancient ecosystems on Earth, whereas the genomes of extant thermophiles place them at the base of the bacterial phylogenetic tree (Reysenbach and Shock 2002).
At the other extreme of temperature, there were two significant glacial episodes during the Precambrian, the first approximately 2.4-2.2 Ga and the second approximately 820-550 Ma. In the most extreme interpretation of these glaciations, the entire surface of the earth was covered in ice - the "Snowball Earth" hypothesis (Kirschvink 1992; Hoffman et al. 1998). Regardless of the controversy surrounding the extent of extreme environments on early Earth, the evidence is clear that they were more widespread than they have been in the more recent past, existing for long intervals during the emergence and early evolution of microbial ecosystems.
1.2.3. Microbial Diversification
Not only have microbes been evolving for approximately 3.5 billion years, the domains of Bacteria and Archaea have established themselves as the major reservoir of diversity of life on Earth. Prokaryotic life has remained small and relatively lacking in structural and behavioral diversity, but by other measures the diversity far exceeds that of animals. Estimates of taxonomic diversity are currently in the millions (Torsvik et al. 2002), although use of the species as the traditional unit of biodiversity does not work well for prokaryotes. Until recently, bacterial species have been characterized through pure culture in the laboratory. The approximately 4,500 species characterized to date are primarily a reflection of the fact that most microbes cannot be cultured under standard laboratory conditions. Amplified sequences from organisms that are unable to be isolated provide a new measure of diversity and a clear indication that most genetic diversity resides in the microbial world (Pace 1997; Nealson and Stahl 1997).
Even the lack of behavioral diversity of prokaryotes is subject to question. On a fine scale, there is great spatial heterogeneity and complexity within the water column and on the solid surfaces where bacterial films and mats form. There is growing evidence that bacteria respond rapidly to orient themselves and maintain positions in steep chemical concentration gradients and that in bacterial communities, symbiotic pairs of bacteria can arrange themselves in characteristic geometric configurations (Fenchel 2002). Responses to physical environmental cues (light, mechanical disturbance, gravity, and magnetic fields) further extend the behavioral diversity of microbial life (Fenchel 1987).
Another measure of prokaryotic diversity is the range of "energy substrates" and metabolic pathways that have evolved. Microbial metabolic activity affects the formation and dissolution of minerals, and in the case of the most important chemical species with which they interact, they become the driving force of biogeochemical cycles (Nealson and Stahl 1997). New and unexpected microbial metabolic pathways are still being discovered, especially in extreme environments.
A further measure of the remarkable diversity of prokaryotes is the range of environments they occupy and their sphere of influence. In addition to populating every habitable environment on Earth (Newman and Banfield 2001), they occupy a vastly broader range of the atmosphere, hydrosphere, and lithosphere than do animals. Microbes have changed Earth beyond the boundaries of the known biosphere via processes that include oxygenic photosynthesis, nitrogen fixation, and carbon sequestration, as well as participating in fundamental abiotic interactions that fall within the scope of geomicrobiology.
1.2.4. Microbes and the Origins of the Eukaryotic Cell
There is general agreement for the proposition that eukaryotes arose through symbiotic incorporation of bacterial chloroplasts and mitochondria (Margulis 1970, 1993) and that symbiosis was an important source of evolutionary innovation in the early history of life (Margulis and Fester 1991). The fossil record is, of course, silent on cellular structure and cannot be used to evaluate alternative hypotheses for the order of assembly of elements of the eukaryotic cell. These range from a hypothetical stepwise incorporation of chloroplasts from cyanobacteria and subsequent incorporation of mitochondria from proteobacteria to a simultaneous merger of symbiotic partners in a microbial mat consortium (Nisbet and Sleep 2001). Evidence of eukaryote origins in the geologic record consists of molecular biomarkers and the morphology of cellular microfossils (e.g., cell shapes and colony forms not seen in bacteria). Rocks dated at 2.7 Ga contain molecular signatures of eukaryote metabolism (Brock et al. 1999), but those sterane geochemical biomarkers record only one eukaryotic property - the ability to make sterol compounds. It is not until 1.8-1.5 Ga that large microfossils, called acritarchs, begin to appear in the fossil record. Acritarchs have spiny spore coats and diverse cell shapes, implying the presence of a dynamic internal cytoskeleton. The "big bang" of eukaryote evolution did not begin until 1 Ga (Knoll 2003).
1.2.5. Microbes and the Oldest Animal Fossils - the Ediacaran Biota
The evolutionary transition from unicellular to multicellular life has occurred multiple times, and there are many theoretical considerations of how and why it might have happened. However, there is no evidence for the environments, selective pressures, or pathways involved in this pivotal step in achieving higher orders of organismal complexity and few empirical studies (but see Boraas et al. 1998; Rainey and Rainey 2003).
Explaining the emergence of animals (metazoans) is perhaps the most familiar problem in the set of unicellular-multicellular transitions. Although there are sedimentary structures more than 1 billion years old that have been interpreted as metazoan trace fossils (Seilacher et al. 1998), convincing traces and body fossils do not make their debut until late in the Proterozoic Eon.
The most striking historical evidence of a fundamental change in the shape of life appears worldwide in the rock record at or soon after 600 Ma in the form of diverse body fossils of the Ediacaran biota (Narbonne 1998). The flat, effectively two-dimensional body plan and unique modular construction of many Ediacaran organisms (Fig. 1.3a) have evoked images of "alien beings here on earth" (Lewin 1983). Although they initially were interpreted as animals and allocated to existing metazoan phyla (Glaessner 1984), there have been numerous subsequent interpretations, including an extinct sister group to metazoans (Buss and Seilacher 1994) and a separate kingdom, the Vendozoa (Seilacher 1989) or Vendobionta (Buss and Seilacher 1994).
Regardless of their placement in the tree of life, the Ediacaran organisms raise the possibility of a very interesting initial experiment in multicellular evolution via symbiosis. Were the first "animals" autotrophs, depending on either photosymbiotic or chemosymbiotic microbial symbionts for their nutrition? McMenamin (1986) hypothesized that Ediacaran organisms housed photosymbionts based on their two-dimensional construction and lack of morphology that could be interpreted as mouth, anus, food-collecting structures, or gut. Seilacher (1989) proposed a chemosymbiotic mode of nutrition, characterizing the body plan as a two-dimensional reaction chamber between oxic and anoxic environments. Both hypotheses are difficult, but perhaps not impossible to test. Runnegar (1992) observed that oxic-anoxic boundaries could well have existed at the sediment-water interface if extensive late Precambrian microbial mats effectively sealed the sediment surface. New studies of enigmatic sedimentary structures in Late Proterozoic rocks show that resistant microbial mats (matground structures) were ubiquitous at the sediment-water interface at this time (Gehling 1999; Hagadorn and Bottjer 1999; Seilacher 1999).
1.3. Late Precambrian and Early Cambrian earliest animal fossils are associated with ancient microbial mats. a. The two-dimensional body plan and modular construction of the enigmatic Dickinsonia, Late Precambrian Ediacaran biota of South Australia, is adapted to life on microbial mats and possibly chemosymbiotic nutritional strategy at ancient oxic-anoxic interfaces. b. Meandering crawling tracks of an unknown animal in Lower Cambrian rocks in the White-Inyo Mountains, California.
Image not available in HTML version |
© Cambridge University Press