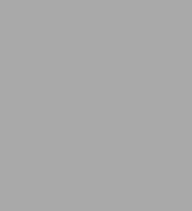
Theory of Linear Poroelasticity with Applications to Geomechanics and Hydrogeology
304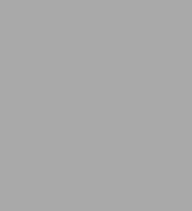
Theory of Linear Poroelasticity with Applications to Geomechanics and Hydrogeology
304eBook
Available on Compatible NOOK devices, the free NOOK App and in My Digital Library.
Related collections and offers
Overview
The theory of linear poroelasticity describes the interaction between mechanical effects and adding or removing fluid from rock. It is critical to the study of such geological phenomena as earthquakes and landslides and is important for numerous engineering projects, including dams, groundwater withdrawal, and petroleum extraction. Now an advanced text synthesizes in one place, with one notation, numerous classical solutions and applications of this highly useful theory.
The introductory chapter recounts parallel developments in geomechanics, hydrogeology, and reservoir engineering that are unified by the tenets of poroelasticity. Next, the theory's constitutive and governing equations and their associated material parameters are described. These equations are then specialized for different simplifying geometries: unbounded problem domains, uniaxial strain, plane strain, radial symmetry, and axisymmetry. Example problems from geomechanics, hydrogeology, and petroleum engineering are incorporated throughout to illustrate poroelastic behavior and solution methods for a wide variety of real-world scenarios. The final chapter provides outlines for finite-element and boundary-element formulations of the field's governing equations. Whether read as a course of study or consulted as a reference by researchers and professionals, this volume's user-friendly presentation makes accessible one of geophysics' most important subjects and will do much to reduce poroelasticity's reputation as difficult to master.
Product Details
ISBN-13: | 9781400885688 |
---|---|
Publisher: | Princeton University Press |
Publication date: | 02/15/2017 |
Series: | Princeton Series in Geophysics , #2 |
Sold by: | Barnes & Noble |
Format: | eBook |
Pages: | 304 |
File size: | 4 MB |
About the Author
Read an Excerpt
Theory of Linear Poroelasticity with Applications to Geomechanics and Hydrogeology
By Herbert F. Wang
Princeton University Press
Copyright © 2000 Princeton University PressAll right reserved.
ISBN: 0-691-03746-9
Chapter One
Introduction1.0 CHAPTER OVERVIEW
The purpose of this book is to quantify, in the simplest linear approximation, how fluid extraction or injection produces stress changes in isotropic, fluid-saturated rock formations; and conversely, how loads applied to an aquifer by engineered structures, atmospheric pressure, earth and ocean tides, tectonic activity, or reservoir lake level changes produce water-level changes in wells. This coupling between changes in stress and changes in fluid pressure forms the subject of poroelasticity. Literal clues to poroelastic phenomena appear in metaphors such as "stressing an aquifer" in reference to pumping a well or "strain meter" in reference to a water well on a fault.
The term poroelasticity was first coined by J. Geertsma as a footnote in his 1966 paper entitled "Problems of rock mechanics in petroleum production engineering." Geertsma's footnote refers specifically to "Biot's work on the theory of the elasticity and viscoelasticity of fluid-saturated porous solids" as "typical of the macroscopic stress-strain relations to be encountered." Geertsma explicitly pointed out that "the mathematical description of the macroscopic theory of poroelasticity is similar to that used in the theory of thermoelasticity." This chapter provides a historical perspective on how the disciplines of geomechanics, hydrogeology, and petroleum engineering contributed to our present understanding of coupled fluid and mechanical behavior.
This chapter previews the constitutive equations of poroelasticity for the case of an isotropic applied stress field. It examines the physical significance of material coefficients such as drained compressibility, poroelastic expansion coefficient, specific storage coefficient, and Skempton's coefficient. The chapter concludes with a description of the analogy between poroelasticity and thermoelasticity.
1.1 HISTORICAL EXAMPLES
Poroelastic behavior can explain an initially unexpected connection between a causal event and its subsequent effect. What follows is a variety of historical examples of poroelastic phenomena:
Water Levels in Wells Correlate with Ocean Tides. Pliny the Elder stated in A.D. 77 that near the temple of Hercules in Cadiz "there is a closed source similar to a well which occasionally rises and falls with the ocean, but at other times does the opposite" (Melchior, 1983, p. 2).
Water Levels Change in Well as Trains Pass. F. H. King (1892) of the University of Wisconsin reported that water levels in a well near the train station at Whitewater, Wisconsin, went up as a train approached and went down as a train left the station. The water level fluctuation was greater for a heavy freight train than for a lighter and faster passenger train.
Water Levels in Boardwalk Wells Fluctuate with Ocean Tides. In 1902 the United States Geological Survey (USGS) reported that water-level oscillations in wells in Atlantic City, New Jersey, were synchronous with ocean depths, because the weight of sea water at high tide compressed the underlying rock, thereby forcing pore water up the wells (Meinzer, 1928).
Texas Claims Oil Fields That Sink into the Sea. The state of Texas claimed title to the part of the Goose Creek oil field near Galveston, Texas, that had become covered with water from Galveston Bay following oil production. The state used the argument that the submerged land belonged to the state. The counterclaim by landowners was based on a geologic study by Pratt and Johnson (1926, p. 582) showing that the subsidence could be attributed to the extraction of 100 million barrels of water, oil, and sand from the reservoir. The courts ruled against the state claim because the submergence was due to human action and not natural causes.
Water Levels Rise in Wells Near a Pumping Well. Observers in the small fishing village of Noordbergum in northern Friesland (Netherlands) witnessed a curious rise in water levels when large pumps were turned on in nearby wells. The behavior lasted a few hours before the water levels dropped. When the large pumps were shut off, the reverse situation occurred-namely, water levels dropped farther before they recovered. Verruijt (1969) concluded that the reverse well fluctuations occurred because pumping instantly compressed the aquifer to force water up the well.
Lake Mead Triggers Earthquakes. The completion in 1935 of Hoover Dam along the Colorado River created 100-meter-deep Lake Mead behind it. Shortly after completion, small earthquakes beneath the lake occurred as a result of a combination of factors (Roeloffs, 1988). One factor was that the additional weight of the dam and water was stressing faults to the failure point; another was that water from the lake seeped into the fault, thereby reducing their resistance to slipping.
1.2 BASIC CONCEPTS
Two basic phenomena underlie poroelastic behavior:
Solid-to-fluid coupling occurs when a change in applied stress produces a change in fluid pressure or fluid mass.
Fluid-to-solid coupling occurs when a change in fluid pressure or fluid mass produces a change in the volume of the porous material.
As used here, the word solid refers to the skeletal framework of bulk, porous material. The terms fluid pressure and pore pressure are also used interchangeably. The solid-to-fluid and fluid-to-solid couplings are assumed to occur instantaneously in the quasistatic approximation in which elastic wave propagation is ignored. The simplest mathematical description of the two basic forms of coupling between solid and fluid is a set of linear constitutive equations. The equations relate strain and fluid-mass changes to stress and fluid-pressure changes. The poroelastic constitutive equations are generalizations of linear elasticity whereby the fluid pressure field is incorporated in a fashion entirely analogous to the manner in which the temperature field is incorporated in thermoelasticity (cf. Section 1.9). An increase of fluid pressure causes the medium to expand just as an increase of temperature causes it to expand.
The magnitude of the solid-to-fluid coupling depends on the compressibility of the framework of the porous material, the compressibility of the pores, the compressibility of the solid grains, the compressibility of the pore fluid, and the porosity. Negligible solid-to-fluid coupling occurs for a highly compressible fluid such as air. An example of solid-to-fluid coupling is the response of water levels in a well to the passage of nearby trains (Fig. 1.1).
Changes of fluid mass or fluid pressure in a porous material produce strains in the bulk, porous solid. A uniform change in fluid pressure throughout a porous body subjected to no external stresses or constraints (free strain case) produces a uniform strain and no poroelastic stresses. In general, consideration of boundary constraints means that the strain field is different from the free strain case, and poroelastic stresses exist within the body. An example of fluid-to-solid coupling is subsidence due to large amounts of fluid extraction from an aquifer or hydrocarbon reservoir (Fig. 1.2).
A nonuniform pore pressure distribution leads to time-dependent fluid flow according to Darcy's (1856) law. The time dependence of pore pressure produces time dependence of poroelastic stresses and strains, which in turn couple back to the pore pressure field. Quantifying these basic poroelastic concepts for application to time-dependent, coupled deformation and fluid-flow problems in hydrogeology, geomechanics, and petroleum engineering is the subject of this book. If only fluid-to-solid coupling were important, the problem would be mathematically simpler because the fluid-flow problem can be solved independently of the stress field. The stress field (and hence strain and displacement fields) could then be calculated as functions of position and time once the pore pressure field has been determined as a function of position and time. This one-way coupling is called the uncoupled problem. However, when the time-dependent changes in stress feed back significantly to the pore pressure, the two-way coupling is important, and the problem is called coupled. In the mathematically analogous subject of thermoelasticity, significant heating of material from stress changes does not occur for most materials, and hence most thermoelastic analyses ignore this direction of coupling, and are uncoupled. However, applied stress changes in fluid-saturated porous materials typically produce significant changes in pore pressure, and this direction of coupling is significant.
1.3 BRIEF HISTORY
Important concepts of poroelasticity developed somewhat independently in geomechanics, petroleum engineering, and hydrogeology. One theme of this book is to highlight the unified description that poroelastic theory provides all three disciplines.
The scientific history of poroelastic concepts spans approximately one hundred fifty years. The early period, from the publication of Darcy's law in 1856 to 1900, included observations of well behavior in response to various loading phenomena such as trains and tides. Increased exploitation of groundwater and hydrocarbon resources in the 1900-1930 period was the impetus for improved scientific and engineering understanding of the principles governing their occurrence and flow. Also, increased civil construction during this period became the impetus for improved understanding of the behavior of soil as a foundation material. The canonical geomechanics problem was soil consolidation, and the canonical hydrogeology problem was elastic storage in a confined aquifer. From 1930 to 1960, significant progress was made in each of the three disciplines in developing fundamental concepts, formulating or extending constitutive laws and governing equations, and obtaining analytical solutions. After 1960, more complex analytical solutions were obtained for problems in land subsidence and earthquake mechanics. Numerical solutions increased in importance as the digital computer enabled more realistic simulations of geological situations and of nonlinear and time-dependent constitutive properties.
1.4 GEOMECHANICS
Karl Terzaghi (1883-1963) sought to understand the behavior of soil as a foundation material by performing controlled laboratory experiments. The work, which led to his consolidation theory, was conducted between 1916 and 1925, when he was assigned by the Austrian Department of Foreign Affairs in Vienna to lecture at Robert University in Istanbul. He published two influential textbooks, Erdbaumechanik auf bodenphysikalischer Grundlage in 1925 and Theoretical Soil Mechanics in 1943. The key experiment from which he developed the governing Eqn. 1.1 is shown schematically in Figure 1.3. A fully saturated soil sample is confined laterally in a cylinder of cross-sectional area A. An axial load - W is applied suddenly at t = 0 and then held constant. (Tensile stresses are taken to be positive.) The water pressure throughout the sample jumps up by the amount p = W/A at t = [0.sup.+]. A profile of excess pressure develops within the sample as water flows out the top drain, which is maintained at atmospheric pressure. Terzaghi derived the consolidation equation for this experiment to be the diffusion equation for excess (greater than hydrostatic) water pressure p,
(1.1) [??] p/[??] t = c [[??].sup.2] p/[??][z.sup.2]
where c is a diffusivity that is known as the consolidation coefficient, t is time, and z is distance along the soil column.
As will be demonstrated in Section 6.3, Eqn. 1.1 is independent of stress, because the theory of poroelasticity leads to the special result that the pore pressure field and applied stress field are uncoupled for the boundary conditions in Terzaghi's experiment. The time evolution of the pressure profile is exactly the same as the analogous thermal conduction problem of a sudden step change (Carslaw and Jaeger, 1959, pp. 96-97), which was noted by Terzaghi.
Terzaghi is generally recognized for elucidating the important concept of effective stress, which for soils is well approximated to be the difference between the applied stress and pore pressure, because the grains are incompressible relative to the bulk soil. Initially, the axial load is borne entirely by the fluid, but it is shifted to the skeletal frame as the excess pore pressure dissipates. A discussion of the effective stress concept is presented in Section 2.8.
1.5 HYDROGEOLOGY
Whereas Terzaghi sought general principles in the laboratory, developments regarding the poroelastic behavior of aquifers were based primarily on field observations. The first published record of water wells responding to passing trains was made by F. H. King (1892) in Wisconsin. He noted that a heavy freight train produced a greater rise in water level than did a lighter and faster passenger train, and that a locomotive alone did not produce a noticeable effect. O. E. Meinzer, in his 1928 paper "Compressibility and elasticity of artesian aquifers," sought to resolve the puzzle of the source of the large amounts of irrigation water pumped from the Dakota sandstone. He reasoned that recharge was insufficient to produce 3000 gallons per minute for 38 years within three townships, and that the compressibility of water alone was likewise insufficient. He concluded that elastic aquifer compression occurred as a result of the decline in fluid pressure, and that the reduction in pore volume was the principal source of water released from storage. He also recognized that aquifers were elastic because well levels recovered after they were shut down. In support of his hypothesis, Meinzer cited King's work on train-induced fluctuations in water levels, the in-phase response of water well levels to ocean tides in Atlantic City, New Jersey (Fig. 1.4), and the subsidence of the Goose Creek oil field. Meinzer also explicitly referenced Terzaghi's effective stress principle to equate pressure decline to an effective stress increase. Meinzer's insight was that he recognized that aquifers were compressible and that the laboratory-derived principle of effective stress could be applied to aquifers. No equations were presented in Meinzer's 1928 paper, although he incorporated calculations for the relative amounts of water released from aquifer compression versus water expansion.
Continues...
Excerpted from Theory of Linear Poroelasticity with Applications to Geomechanics and Hydrogeology by Herbert F. Wang Copyright © 2000 by Princeton University Press. Excerpted by permission.
All rights reserved. No part of this excerpt may be reproduced or reprinted without permission in writing from the publisher.
Excerpts are provided by Dial-A-Book Inc. solely for the personal use of visitors to this web site.
Table of Contents
Preface xiChapter 1: Introduction 3
1.0 Chapter Overview 3
1.1 Historical Examples 3
1.2 Basic Concepts 5
1.3 Brief History 8
1.4 Geomechanics 8
1.5 Hydrogeology 10
1.6 Petroleum Engineering 13
1.7 Biot's Theory 14
1.8 Preview of Constitutive Relations 17
1.9 Thermoelastic Analogy 22
Chapter 2: Linear Constitutive Equations 26
2.0 Chapter Overview 26
2.1 Kinematic Variables 26
2.2 Dynamic Variables 30
2.3 Constitutive Relations 31
2.4 Principal Coordinates 33
2.5 Isotropic Stress and Strain 34
2.6 Nonprincipal Coordinates 37
2.7 Anisotropy 42
2.8 Effective Stress 43
3. Poroelastic Constants 47
Chapter 3: Chapter Overview 47
3.1 Compressibility 47
3.2 Drained versus Undrained Moduli 53
3.3 Storage Capacity 55
3.4 Hydraulic Diffusivity 61
3.5 Poroelastic Expansion Coefficients 62
3.6 Coefficients of Undrained Pore-Pressure Buildup 64
3.7 Incompressible or Highly Compressible Constituents 68
3.8 Laboratory Data 69
Chapter 4: Governing Equations 71
4.0 Chapter Overview 71
4.1 Force Equilibrium Equations 72
4.2 Displacement Formulation 73
4.3 Beltrami-Michell Equations 76
4.4 Strain Compatibility Equations 77
4.5 Mechanical Boundary and Initial Conditions 78
4.6 Darcy's Law 79
4.7 Fluid Continuity 83
4.8 Comparison of Diffusion Equations 86
4.9 Fluid Boundary and Initial Conditions 87
4.10 Uncoupling of Stress or Strain from Pore Pressure 88
4.11 Force Equilibrium for the Solid Matrix 91
Chapter 5: Unbounded Problem Domains 96
5.0 Chapter Overview 96
5.1 Poroelastic Displacement Potential in Infinite Domain 96
5.2 Uniform Fluid Injection in a Sphere 98
5.3 Green's Functions 101
5.4 Center of Dilatation 103
5.5 Half Space with Traction-Free Boundary 105
5.6 Gravity Analogy 107
5.7 Sudden Injection of Fluid at a Point in Infinite Domain 110
5.8 Fluid Dipole Equivalence to Body-Force Distributions 112
Chapter 6: Uniaxial Strain 116
6.0 Chapter Overview 116
6.1 Constitutive Equations 116
6.2 Force Equilibrium Equation 118
6.3 Fluid Diffusion Equation 119
6.4 Step Load on Semi-Infinite Column 120
6.5 Consolidation of a Finite Layer (Terzaghi's Problem) 124
6.6 Uniformly Increasing Load on a Finite Layer 129
6.7 Sedimentation on Impermeable Basement 131
6.8 Erosional Unloading 134
6.9 Periodic Load on a Half Space 136
6.10 Periodic Load on a Finite Layer 138
6.11 Water Load on a Half Space 140
Chapter 7: Plane Strain and Plane Stress in Rectangular Coordinates 150
7.0 Chapter Overview 150
7.1 Constitutive Equations for Plane Strain 151
7.2 Governing Equations for Plane Strain 151
7.3 Mandel's Problem 153
7.4 Biot's Stress Function 157
7.5 Periodic Load on a Half Space 158
7.6 Displacement Functions 163
7.7 Sudden Step Load on a Half Space 165
7.8 Undrained Response to a Line Source 170
7.9 Sudden Fault Slip 172
7.10 Constitutive Equations for Plane Stress 183
8. Plane Strain in Polar Coordinates 187
Chapter 8: Chapter Overview 187
8.1 Radial Symmetry 187
8.2 Sudden Pressurization of a Long Cylinder 191
8.3 Sudden Pressurization of a Borehole 201
8.4 Sudden Stress Release due to Borehole Excavation in an Anisotropic Stress Field 203
8.5 Hydraulic Fracturing 205
8.6 Sudden Internal Pressurization of a Hollow Cylinder 206
8.7 Line Source in Plane Strain 211
Chapter 9: Axisymmetry 215
9.0 Chapter Overview 215
9.1 Governing Equations 215
i 9.2 Pumping from a Well 216
9.3 Reverse Water-Level Fluctuations 218
9.4 Pulse-Decay Test 221
9.5 Elastostatic Subsidence of a Half Space 226
9.6 Time-Dependent Subsidence of a Half Space 229
Chapter 10: Numerical Methods 238
10.0 Chapter Overview 238
10.1 Finite-Element Method 238
10.2 Boundary-Element Method 245
Appendix A: Integral Transforms 257
A.1 Properties of Fourier Transforms 257
A.2 Properties of Laplace Transforms 258
A.3 Stehfest Algorithm for Laplace Transform Inversion 259
A.4 Properties of Hankel Transforms 260
Appendix B: Relations Among Poroelastic Constants 262
Appendix C: Representative Poroelastic Constants 265
Symbols 267
References 269
Uniited References 279
Author Index 283
Subject Index 286
What People are Saying About This
"This book is a significant contribution to the poroelasticity field and one which might well become a classic. It is an invaluable resource for hydrogeologists and geotechnical and petroleum engineers. It can also be easily adopted as a graduate-level texbook. The scholarship is sound. The content is effectively organized and well written. I strongly recommend it."—Shemin Ge, University of Colorado, Boulder
This book is a significant contribution to the poroelasticity field and one which might well become a classic. It is an invaluable resource for hydrogeologists and geotechnical and petroleum engineers. It can also be easily adopted as a graduate-level texbook. The scholarship is sound. The content is effectively organized and well written. I strongly recommend it.
Shemin Ge, University of Colorado, Boulder
Recipe
"This book is a significant contribution to the poroelasticity field and one which might well become a classic. It is an invaluable resource for hydrogeologists and geotechnical and petroleum engineers. It can also be easily adopted as a graduate-level texbook. The scholarship is sound. The content is effectively organized and well written. I strongly recommend it."Shemin Ge, University of Colorado, Boulder