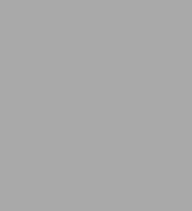
Trophic Cascades: Predators, Prey, and the Changing Dynamics of Nature
488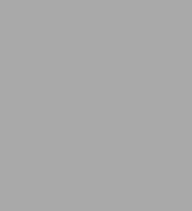
Trophic Cascades: Predators, Prey, and the Changing Dynamics of Nature
488eBook
Available on Compatible NOOK devices, the free NOOK App and in My Digital Library.
Related collections and offers
Overview
Trophic Cascades is the first comprehensive presentation of the science on this subject. It brings together some of the world’s leading scientists and researchers to explain the importance of large animals in regulating ecosystems, and to relate that scientific knowledge to practical conservation.
Chapters examine trophic cascades across the world’s major biomes, including intertidal habitats, coastal oceans, lakes, nearshore ecosystems, open oceans, tropical forests, boreal and temperate ecosystems, low arctic scrubland, savannas, and islands. Additional chapters consider aboveground/belowground linkages, predation and ecosystem processes, consumer control by megafauna and fire, and alternative states in ecosystems. An introductory chapter offers a concise overview of trophic cascades, while concluding chapters consider theoretical perspectives and comparative issues.
Trophic Cascades provides a scientific basis and justification for the idea that large predators and top-down forcing must be considered in conservation strategies, alongside factors such as habitat preservation and invasive species. It is a groundbreaking work for scientists and managers involved with biodiversity conservation and protection.
Product Details
ISBN-13: | 9781597268196 |
---|---|
Publisher: | Island Press |
Publication date: | 06/25/2013 |
Sold by: | Barnes & Noble |
Format: | eBook |
Pages: | 488 |
File size: | 28 MB |
Note: | This product may take a few minutes to download. |
About the Author
John Terborgh is James B. Duke Professor of Environmental Sciences and codirector of the Center for Tropical Conservation at Duke University. He has devoted much of the past 35 years to issues concerning the ecology and conservation of neotropical systems.
After spending most of his career as a research scientist with the US Geological Survey, James A. Estes is currently a faculty member at the University of California at Santa Cruz where he holds a position of professor of ecology and evolutionary biology.
Read an Excerpt
Trophic Cascades
Predators, Prey, and the Changing Dynamics of Nature
By John Terborgh, James A Estes
ISLAND PRESS
Copyright © 2010 Island PressAll rights reserved.
ISBN: 978-1-59726-819-6
CHAPTER 1
Trophic Cascades: What They Are, How They Work, and Why They Matter
John Terborgh, Robert D. Holt, and James A. Estes
Humans have been waging war against predators since the dawn of history. Lion slayers were heroes of Greek mythology. Shepherds bred large, aggressive dogs to fend off wolves and bears in the Pyrenees, Carpathians, and elsewhere. Gamekeepers were hired by the great estates of Britain to eradicate foxes, goshawks, and badgers. In the United States, an agency of the federal government, the Biological Survey (later the U.S. Department of the Interior), hired hundreds of predator control agents to shoot, trap, and poison wolves, cougars, coyotes, eagles, and a host of lesser predators. Bounties and culls have been used in Alaska and Canada to control seals and sea lions in the name of fishery management. An almost endless list of such measures could be compiled.
Humans have been so effective at decimating or entirely eliminating predators over most of the land and sea that the effects of these persecutions are becoming apparent to the ordinary citizen. There is hardly a resident of suburban America today whose efforts to grow flowers or vegetables isn't thwarted by ubiquitous deer. Ask any gardener why there are so many deer, and the answer is a consistent refrain: "Because they don't have any predators." The line of reasoning from cause to effect is simple and linear. It is precisely what Hairston, Smith, and Slobodkin (HSS 1960) posited nearly 50 years ago.
Recognition on the part of official agencies that predators play important roles in nature has been belated but is now spreading in the United States, Canada, Europe, and the industrialized countries generally, where legal structures protect wildlife and managers intervene to mitigate human–wildlife conflicts. In these countries, the tide of opinion is changing. Whereas predators were actively persecuted a generation ago, they are now being restored. Examples from the United States include reintroduction of the gray wolf in Wyoming, Mexican wolf in Arizona and New Mexico, red wolf in North Carolina, lynx in Colorado, black-footed ferret in Wyoming, South Dakota, and Chihuahua, Mexico, and sea otters in southeast Alaska, Washington, and southern California. And after an absence of more than 100 years, jaguars are returning to the borderlands between the United States and Mexico.
Why should we celebrate this development and encourage its expansion to additional places and predators in other parts of the world? The answer is a complicated one, blending philosophical, aesthetic, practical, and scientific reasoning. In this book we shall be concerned primarily with the scientific reasons for sustaining predators while recognizing that more philosophical approaches are also valid.
Predators are important because they occupy the top rung of the trophic ladder and from that position regulate the food web below them. Top vertebrate predators are large bodied and can move over large areas, thus coupling the dynamics of seemingly distinct communities and ecosystems. Recently, the ability of predators to move flexibly between communities, responding opportunistically to shifts in prey abundance, has been suggested to be an important governor of food web stability (McCann et al. 2005; Holt 2009). Eliminating predators destabilizes ecosystems, setting off chain reactions that eventually cascade down the trophic ladder to the lowest rung. In 1980, Robert Paine coined the term trophic cascade to describe this process. The altered state that develops after the loss of apex predators is invariably simpler than the initial state, supporting less biodiversity. Thus, predators hold an important key to retaining the high levels of biodiversity we associate with primordial nature.
Three-level cascades are the simplest and most familiar case (e.g., wolf–deer–vegetation). Wolves eat deer and thereby indirectly benefit vegetation, depending on their efficiency in maintaining deer numbers at low levels. If wolves are efficient deer predators, deer populations remain low and the vegetation experiences only light herbivory; if they are inefficient, deer populations are higher and herbivory is heavier. This efficiency is analogous to Paine's (1980) interaction strength. However, unlike the case of Paine's Pisaster, it is generally not possible to use rigorous, controlled experiments to determine the impact of a large predator such as a wolf because such demonstrations require wolf removal and subsequent assessment of the demographic response of deer. Responses of deer and their allies to the local extirpation of wolves or other top predators have typically lagged by decades, during which time other factors, such as plant succession, hunting, land use changes, and other human activities, can intervene to complicate the picture (McShea et al. 1997).
The simple example of the wolf–deer–vegetation interaction introduces some key features of trophic cascades. First, predators harvest prey with a certain efficiency that can vary with the topography, vegetation, density, and evasive behavior of the prey and perhaps other factors such as other species of prey and predators (Berger 2008). Thus, the strength of the top-down interaction is not a simple property of predator and prey alone, but it depends on the context in which the interaction takes place. Second, we note that the wolf population interacts only with deer; it is at the top of the pyramid and regulated from the bottom up via the deer population that supports it. However, the deer population is in the middle of a bidirectional flow of resources. It depends on forage (a bottom-up process) and is preyed on by wolves (a top-down process).The density of the deer population thus depends on the balance of these two countercurrent forces. Finally, the vegetation is also regulated both by bottom-up (water, sunlight, nutrients) and by top-down (herbivory) processes. In essence, this simple bidirectional interaction scheme is what Hairston, Smith, and Slobodkin proposed in 1960 in their famous "green world" hypothesis.
A common misimpression is that there is an either–or dichotomy between systems driven from the bottom up and those driven from the top down. Bottom-up and top-down processes are not in any way exclusive; they are complementary countercurrent flows, inextricably bound together. Bottom-up processes are fundamental and inescapable, driving photosynthesis and being supported by it. If photosynthesis increases, as along a climatic gradient for example, the responses are quantitative: more productivity, more herbivores, more predators being supported by those herbivores, and occasionally an increase in food chain length (Crête 1999). Only at the very lowest productivity levels, such as near the limits of vegetation in deserts or the high Arctic, does one find ecosystems with fewer than the three standard levels (Oksanen et al. 1981).
With the exception of microbial ecosystems supported by chemoautotrophs, photosynthetic productivity determines the availability of resources to higher levels, either directly (via chains starting with herbivory) or indirectly via detritivores (e.g., the deep sea, caves). How primary productivity is allocated among higher levels is determined not only by the efficiency of material and energy flux upward through the food chain but also from the top down through the trophic cascade. In the absence of herbivores or predators, the entire annual net productivity of a patch of vegetation must pass into the detrital food web. If herbivores are present, some of the productivity will accrue to them and less will recycle through detritus. If predators are added, the flow of resources will ascend one level further, and less may accrue to herbivores because their numbers are kept in check by the predators (although they will probably turn over faster). Thus, bottom-up processes determine the flow of resources into the system, whereas top-down processes influence how the resources are distributed among trophic levels.
Another issue that has led to confusion is whether trophic cascades are inherently static or dynamic. In fact, a trophic cascade is always dynamic, but the dynamism is not always manifest. When a trophic system is at a stable point (i.e., equilibrium), its component levels remain fairly constant. However, the appearance of stasis is illusory, suggesting the absence of any dynamic process. But under the surface, the interactions between levels are not static but rather highly dynamic. Predators are eating prey, prey are eating plants, plants are growing, and so on. A lot is happening, but the various interacting forces and flows are in balance, so the underlying dynamism is not apparent. Call it cryptic dynamism. The stable, equilibrial condition is quite properly called a trophic cascade because the term refers to the whole interacting system, not just to one or another of the states it can assume.
A final point that warrants clarification in these preliminary comments concerns the role of keystone species in trophic cascades. Paine's (1966) founding example of the starfish Pisaster has left an indelible mark on the literature. Pisaster is an unequivocal keystone species, defined by Mary Power and colleagues (1996) as one having effects on other elements of an ecosystem that are large relative to its numbers or biomass. The wolves of Yellowstone are another keystone species. Dramatic responses can be obtained by perturbing a keystone species, but are keystone species necessary as mediators of strong trophic cascades? The Pisaster example, and some other equally dramatic ones, has led some authors to conclude that keystone species are necessary ingredients of strong trophic cascades (Polis et al. 2000). We shall see definitively that this is not the case. Keystone species are notable because they concentrate much of the interaction strength of an entire trophic level in a single species, but across nature more generally, keystone species possessing such concentrated interaction strength are probably the exception rather than the rule.
Because of controversy over why the world is green, there has been a focus in much of the trophic cascade literature on indirect carnivore impacts on plants (or space occupiers in marine systems), via shifts in herbivore abundance and activity. The concept of a trophic cascade actually has a much broader scope than just indirect mutualisms between predators and plants; the basal species might be space occupiers in marine systems or detritivores and decomposers in soil food webs, for instance. There can also be trophic cascades between species, all of which are predators (e.g., in the Bahamas, lizard cuckoos may eat Anolis lizards or force them into hiding and so reduce predation on spiders). But the main heat in the literature on trophic cascades seems to arise from efforts to understand patterns in plant communities.
The basic question posed by HSS (and, they believed, answered by them) is this: To understand plant ecology (e.g., distributions of life forms within a community, or the distribution of plant species along environmental gradients), must one pay attention to the food webs supported by those plant communities? It is fair to say that plant ecology has traditionally focused on how plant form, life history, and species composition reflect the outcome of competitive interactions and population dynamics playing out in the context of various factors in the physical environment (e.g., climate, soil, disturbance regimes). This unilaterally bottom-up view of plant ecology essentially ignores herbivory as a deterministic force in structuring vegetation. But if trophic cascades are ubiquitous and large, as we are convinced, bottom-up forcing is only half the picture.
Some authors argue that trophic cascades are idiosyncratic in occurrence and not all that important. For instance, Polis et al. (2000) suggest that in contrast to aquatic systems, "community cascades ... are absent or rare in terrestrial habitats" (473); furthermore, they claim that "support for even species-level cascades is limited in terrestrial systems" (474).
The reasons they give for these assertions are that most food webs have a reticulate and heterogeneous structure and that many prey, plants in particular, are inedible.
Biological control of agricultural pests is a widespread application of trophic cascades to solve practical problems in applied terrestrial ecology, so the second quote from Polis et al., taken literally, is false. But agricultural systems, by design, tend to be low in species diversity and other kinds of heterogeneity, and crop plants have been bred to be edible (at least to us) at the expense of spines, secondary compounds, low-quality tissues, and other antiherbivore devices. So maybe biological control is the exception that proves the rule: Trophic cascades may be ubiquitous in the artificial landscapes of agroecosystems without being significant drivers of plant community structure and dynamics in natural ecosystems.
Our reading of the literature suggests that the claims of Polis et al. (2000) are greatly overstated. This volume is replete with convincing evidence of cascades in terrestrial and aquatic systems. Moreover, it should be noted that there are limitations in most experimental studies of trophic cascades in terrestrial biomes (Holt 2000). For understandable reasons, most manipulative studies are short term. For instance, in the review by Schmitz et al. (2000), 80 percent of the studies involved measurements over a single growing season, even though many of the target species were long-lived shrubs, trees, perennial herbs, and graminoids. The time scales of transient dynamics in trophic cascades in terrestrial systems are likely to be much longer than in many aquatic systems, where the basal producers (e.g., phytoplankton) have short generation times and so can respond very rapidly to shifts in herbivory. A small quantitative impact of herbivory observed in a single growing season in a terrestrial system that seems quite subtle, assessed, say, in terms of individual growth rates or tissue damage, could be magnified over time, for instance, by altering competitive ability. Shifts in community composition caused by altered herbivory regimes could necessitate colonization from a regional source pool, followed by shifts in local abundances, both of which could be very slow processes, especially if the vegetation is woody. Patterns in abundance as a function of trophic level along gradients reveal the importance of such within-level species sorting for elucidating natural patterns (Leibold 1996; Leibold et al. 1997). Finally, manipulative studies never remove all the natural enemies of herbivores. These include not just predators but parasitoids, pathogens, entomophagous nematodes, and so on. Experiments rarely run long enough so that the regional species pool of potentially important herbivores is sampled at an experimental site.
(Continues...)
Excerpted from Trophic Cascades by John Terborgh, James A Estes. Copyright © 2010 Island Press. Excerpted by permission of ISLAND PRESS.
All rights reserved. No part of this excerpt may be reproduced or reprinted without permission in writing from the publisher.
Excerpts are provided by Dial-A-Book Inc. solely for the personal use of visitors to this web site.
Table of Contents
Foreword\Ellen K. PikitchPreface
Chapter 1. Trophic Cascades: What They Are, How They Work, and Why They Matter\John Terborgh, Robert D. Holt, and James A. Estes
PART I. Aquatic Ecosystems
Chapter 2. Food Chain Dynamics and Trophic Cascades in Intertidal Habitats\Robert T. Paine
Chapter 3. Some Effects of Apex Predators in Higher-Latitude Coastal Oceans\James A. Estes, Charles H. Peterson, and Robert S. Steneck
Chapter 4. Trophic Cascades in Lakes: Lessons and Prospects\Stephen R. Carpenter, Jonathan J. Cole, James F. Kitchell, and Michael L. Pace
Chapter 5. Prey Release,Trophic Cascades, and Phase Shifts in Tropical Nearshore Ecosystems\Stuart A. Sandin, Sheila M.Walsh, and Jeremy B. C. Jackson
Chapter 6. Trophic Cascades in Open Ocean Ecosystems\Tim Essington
PART II. Terrestrial Ecosystems
Chapter 7. The Role of Herbivores in Terrestrial Trophic Cascades\Robert J. Marquis
Chapter 8. Propagation of Trophic Cascades via Multiple Pathways in Tropical Forests\John Terborgh and Kenneth Feeley
Chapter 9. Large Predators, Deer, and Trophic Cascades in Boreal and Temperate Ecosystems\William J. Ripple,Thomas P. Rooney, and Robert L. Beschta
Chapter 10. Islands as Tests of the Green World Hypothesis\Lauri Oksanen,Tarja Oksanen, Jonas Dahlgren, Peter Hambäck, Per Ekerholm, Åsa Lindgren, and Johan Olofsson
Chapter 11. Trophic Cascades on Islands\Thomas W. Schoener and David A. Spiller
Chapter 12. Trophic Cascades,Aboveground–Belowground Linkages, and Ecosystem Functioning\David A.Wardle
PART III. Predation and Ecosystem Processes
Chapter 13. Ecological and Conservation Implications of Mesopredator Release\Justin S. Brashares, Laura R. Prugh, Chantal J. Stoner, and Clinton W. Epps
Chapter 14. Fear-Mediated Food Webs\Joel Berger
Chapter 15. Trophic Cascades in African Savanna: Serengeti as a Case Study\A. R. E. Sinclair, Kristine Metzger, Justin S. Brashares,Ally Nkwabi, Gregor Sharam, and John M. Fryxell
Chapter 16. Consumer Control by Megafauna and Fire\William Bond
Chapter 17. Alternative States in Ecosystems\Marten Scheffer
PART IV. Synthesis
Chapter 18. Theoretical Perspectives on Trophic Cascades: Current Trends and Future Directions\Robert D. Holt, Ricardo M. Holdo, and F. J. Frank van Veen
Chapter 19. Comparing Trophic Cascades across Ecosystems\Jonathan B. Shurin, Russell W. Markel, and Blake Matthews
Chapter 20. Conservation Relevance of Ecological Cascades\Michael E. Soulé
Chapter 21. Conclusion: Our Trophically Degraded Planet\John Terborgh and James A. Estes
References
Contributors
Index