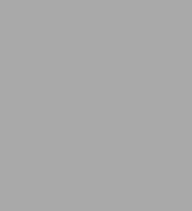
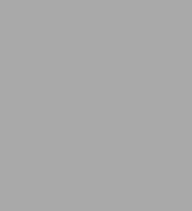
eBook
Available on Compatible NOOK devices, the free NOOK App and in My Digital Library.
Related collections and offers
Overview
The revised and updated fourth edition of Understanding Lasers offers an essential guide and introduction that explores how lasers work, what they do, and how they are applied in the real world. The author—a Fellow of The Optical Society—reviews the key concepts of physics and optics that are essential for understanding lasers and explains how lasers operate. The book also contains information on the optical accessories used with lasers.
Written in non-technical terms, the book gives an overview of the wide-variety laser types and configurations. Understanding Lasers covers fiber, solid-state, excimer, helium-neon, carbon dioxide, free-electron lasers, and more. In addition, the book also explains concepts such as the difference between laser oscillation and amplification, the importance of laser gain, and tunable lasers. The updated fourth edition highlights the most recent research and development in the field. This important resource:
- Includes a new chapter on fiber lasers and amplifiers
- Reviews new topics on physics of optical fibers and fiber lasers, disk lasers, and Ytterbium lasers
- Contains new sections on Laser Geometry and Implications, Diode Laser Structures, Optimal Parametric Sources, and 3D Printing and Additive Manufacturing
- Puts the focus on research and emerging developments in areas such as spectroscopy, slow light, laser cooling, and extremely precise measurements
- Contains appendices, glossary, and index that help make this book a useful reference
Written for engineering and physics students, engineers, scientists, and technicians, the fourth edition of Understanding Lasers contains the basic concepts of lasers and the most recent advances in the technology.
Product Details
ISBN-13: | 9781119310662 |
---|---|
Publisher: | Wiley |
Publication date: | 11/27/2018 |
Sold by: | JOHN WILEY & SONS |
Format: | eBook |
Pages: | 608 |
File size: | 8 MB |
About the Author
Read an Excerpt
Understanding Lasers
An Entry-Level GuideBy Jeff Hecht
John Wiley & Sons
ISBN: 0-7803-1005-5Chapter One
Introduction & Overview
ABOUT THIS CHAPTER
This chapter will introduce you to lasers. It will give you a basic idea of how they work, how they are used, and what their important properties are. This basic understanding will serve as a foundation for the more detailed descriptions of lasers in later chapters.
LASERS IN FACT AND FICTION
The laser sits near the top of any list of the greatest inventions of the last half of the twentieth century. Together with the satellite, the computer, and the integrated circuit, it is a symbol of "high technology." Like the other technologies, lasers affect our lives in many ways, and are growing steadily in importance. Laser technology is both fascinating in itself and an important tool in fields from medicine to communications. However, few people outside the field understand it well.
Arthur Schawlow posted his sign distinguishing the "incredible" lasers of public misconception from the "credible" lasers of his laboratory in 1962. Over the years, he has given away many copies of the poster, and it has amused many students, laser engineers and scientists. Yet the basic point remains true. Too many people confuse real lasers with science-fictional ray guns or death rays. Military researchers have spent immense sums trying to develop laser weapons, but so far none have reached the battlefield. After years of research, the Pentagon backed away from plans to use high-power lasers to defend against nuclear attack. The only realistic uses of laser weapons in the near future are to attack the targets most vulnerable to light-electro-optical sensors and the human eye.
Real laser technology may not be as spectacular, but it is important and fascinating. Lasers can send signals through miles of fiberoptic cable, print computer output, read printed codes in the supermarket, diagnose and treat disease, cut and weld materials, and make ultraprecise measurements. With laser light you can record three-dimensional holograms, project bright light-show images, spot flaws in a centuries-old painting, or play crystal-clear digital music recorded on a compact disc. This book will teach you how this real laser technology works.
WHAT IS A LASER?
The word laser was coined as an acronym, for light amplification by the stimulated emission of radiation, and those words have special meanings. They tell us that laser light is special light. Ordinary light, from the sun or a light bulb, is emitted spontaneously, when atoms or molecules release excess energy by themselves, without any outside intervention. Stimulated emission is different, because it occurs when an atom or molecule that is holding excess energy is "stimulated" to emit that energy as light. Well come back to look more closely at stimulated emission in Chapter 3.
Albert Einstein first suggested the possibility of stimulated emission in a paper published in 1917. However, for many years physicists thought that atoms and molecules always were much more likely to emit light spontaneously, and that stimulated emission thus always would be much weaker. It was not until after World War II that physicists began trying to make stimulated emission dominate. They sought ways for one atom or molecule to stimulate many others to emit light, amplifying it to much higher powers.
The first to succeed was Charles H. Townes, then at Columbia University. He did not work with light at first, but with microwaves at much longer wavelengths, building a device he called a maser, for microwave amplification by the stimulated emission of radiation. He thought of the key idea in 1951, but the first maser was not completed until a couple of years later. Before long, many other physicists were building masers, and trying to find how to stimulate emission at even shorter wavelengths.
The key concepts emerged about 1957. Townes and Arthur Schawlow, then at Bell Telephone Laboratories, wrote a long paper outlining the conditions needed to amplify stimulated emission of visible light waves. At about the same time, similar ideas crystallized in the mind of Gordon Gould, then a 37-year-old graduate student at Columbia, who wrote them down in a series of notebooks. Townes and Schawlow published their ideas in a scientific journal, Physical Review Letters, but Gould filed a patent application.
Well over decades later, people still argue about who deserves credit for the laser concept. Townes and two Soviet maser pioneers, Nikolai Basov and Aleksander Prokhorov, shared the 1964 Nobel Prize in Physics for their pioneering work on "the maser/laser principle." Schawlow eventually shared the 1981 Nobel Physics Prize for research done with lasers. For many years, Gould had to settle only for credit for coining the word "laser" in his notebooks. However, after nearly two decades of legal struggles and comparative obscurity, he finally received four patents worth millions of dollars on important aspects of laser technology. The last of those patents was issued in May 1988, over 30 years after Gould sat down to write his notebooks.
Publication of the paper by Townes and Schawlow stimulated many efforts to make lasers. Schawlow and others went to work at Bell Laboratories. Gould took his ideas to a small Long Island company, TRG Inc., which used them in a research proposal to the Department of Defense. The Pentagon brass was enthusiastic about the idea, but not about Gould's brief involvement with a Marxist study group a decade earlier, so they wouldn't grant Gould the security clearance he needed to work on the project. Scientists at many other government, industry and university laboratories also tried to make lasers.
The winner of the great laser race, on May 16, 1960, was a dark horse, Theodore Maiman. He had decided that synthetic ruby was a good laser material, even though Schawlow had publicly said it wouldn't work. Maiman's managers at Hughes Research Laboratories in Malibu, California had told him to stop wasting his time trying to make a ruby laser. But Maiman continued, and by putting mirrors on each end of a ruby rod and illuminating it with a bright flashlamp, he made the world's first laser. A replica is shown in Fig. 1-1.
Many other types of lasers followed, but Maiman's ruby laser was typical in many ways of what we expect a laser to be. It emitted light in a narrow, tightly concentrated beam. The light was at a single wavelength-694 nanometers (1 nm = [10.sup.-9] meter) at the red end of the visible spectrum. The light waves were coherent, all aligned with each other and marching along in step.
Maiman's laser emitted light in short, intense pulses, and many other lasers also operate in pulsed mode. However, other lasers emit steady beams. Power levels can span a wide range. Some lasers emit less than a milliwatt (0.001 watt) of light; others emit steady beams of many kilowatts. The highest laser powers-trillions of watts-are achieved in ultrashort pulses lasting only about a billionth of a second. Figure 1-2 shows a sampling of commercial lasers.
We think of lasers as emitting visible light, but the basic principles behind the laser are valid at many other wavelengths. We already saw that Townes produced the first stimulated emission of microwaves. Many lasers emit infrared and ultraviolet light, and researchers now are working on X-ray lasers.
Maiman's first laser was small enough for him to hold in one hand, but modern lasers come in many different sizes. The most common lasers are semiconductor types, the size of a grain of salt, used in compact disc digital audio players and fiber-optic communications. Government laboratories have built monstrous lasers that fill the interiors of good-sized buildings. Other lasers come in many sizes between, including pen-sized laser pointers, benchtop laboratory lasers, and materials-working lasers packaged so they look like heavy machine tools.
Well learn more about these properties of lasers in later chapters. For now, it is most important to remember that the types of lasers are many and varied.
HOW LASERS ARE USED
In the early 1960s, it seemed that every physicist and engineer wanted to get his or her hands on a laser and play with it. They aimed lasers at just about everything they could find. They shot so many holes in razor blades that for a while laser power was informally measured in "gillettes." Yet there were few practical applications, and for a time the laser seemed to be, in the words of one wag, "a solution looking for a problem."
That is no longer true. Lasers are now used for many purposes in many fields. A sampling of laser applications is listed in Table 1-1; Chapters 10 and 11 describe some of those uses in more detail.
We can get a better feeling for how lasers are used by breaking down major applications into families. One large family of applications uses lasers as highly directional light bulbs for information processing, such as reading, writing, or sending signals. Another uses lasers as precise light sources for a wide variety of measurements. Another puts laser light to work for medical treatment. Laser energy also can be used to change things, from marking symbols on parts to welding thick sheets of steel. Military agencies use lasers for weapon control and simulation, and are studying lasers for use as weapons. Other applications rely on other special properties of laser light, such as the way its light waves are aligned, or the fact that it consists of only a single color.
Some low-power lasers may seem to do the same job as a light bulb, but they can do it better. For example, supermarkets use laser scanners under checkout counters to read bar codes on packages. The bar code identifies each product to the store's computer, which recalls the price and prints it out at the terminal. Why use a laser? The red laser light is in a thin, highly directional beam, so it can be focused onto packages at various distances above the counter. The scanner includes a filter that cuts out all light other than the single shade of red emitted by the laser, reducing the likelihood of errors caused by overhead lights. Engineers looked at many other alternatives, and decided the laser was best for supermarket checkout. Non-laser light sources may be used when hand-held wands are run directly over the bar code on the surface, keeping out stray light.
Semiconductor lasers are widely used because they are very small and can produce a few milliwatts of light that can be tightly focused to a very small spot. That is ideal for many uses, ranging from playing music on compact discs to sending signals through optical fibers. What's more, by varying the current passing through the laser, you can control how much light it emits-ideal for communications or writing computer output.
Other lasers produce short, intense pulses of light. If these pulses are focused, they can concentrate a lot of energy on a tiny spot. Such pulses can make tiny marks on a metal surface, or even drill holes, particularly if a series of pulses is focused onto the same spot. Because laser pulses do not get dull, they are very good for drilling hard materials like titanium metal or even diamond. They are also very good for drilling into soft objects like rubber or many plastics, because they don't twist, bend or turn the material. A series of pulses, or a higher-power steady beam, can cut or weld. Physicians can carefully control laser energy to remove diseased tissue without damaging healthy cells. Computers can control cutting and drilling lasers in automated machining centers.
The alignment of laser light waves that makes it coherent is ideal for holography, the recording and reconstruction of three-dimensional images. Holograms make striking artistic displays, projecting three-dimensional images that look so real you want to reach out and touch them. They also serve more practical purposes. Holograms are hard to make without special equipment, so they are placed on credit cards to show that the cards are genuine. You can measure tiny deformations in an object by recording two holograms on the same piece of film, one before a stress is applied and one after. Illuminate the film to produce an image, and you can see where the object moved. This lets inspectors spot flaws in aircraft tires before they are put on a plane, so you can ride more safely.
In later chapters, we will delve more into laser applications. We will also see that new applications are being developed steadily. Government and industrial laboratories are working on laser techniques for tasks ranging from treating cancer to controlling nuclear fusion.
IMPORTANT LASER PROPERTIES
Lasers take many different forms and have many differing characteristics, such as their output wavelength and power level. However, different types of lasers often share some common properties, such as the concentration of their output energy in a narrow beam. We list many important properties of lasers below. Each deserves a bit more explanation.
MAJOR LASER PROPERTIES
Wavelength(s)
Output power
Duration of emission (pulsed or continuous)
Beam divergence and size
Coherence
Efficiency and power requirements
Wavelength(s)
As we will see in Chapter 2, light is made of waves, and the length of those waves, or the wavelength, is a fundamental characteristic of the light. We sense the wavelength of visible light as color (although that sensing is not very precise). Each type of laser emits a characteristic wavelength or a small range of wavelengths. The wavelengths depend on the type of material that emits the laser light, the laser's optical system, and the way the laser is energized. Laser action can produce infrared, visible and ultraviolet light; "masers" produce microwaves by essentially the same process. If you want a particular wavelength, your choice is usually limited to no more than a few types of lasers. Table 1-2 lists a sampling of important laser types and their wavelengths in nanometers.
Most lasers are called monochromatic (single-colored) and nominally emit only one wavelength. Actually, they emit a range of wavelengths, but the range is narrow enough to be considered a single wavelength for most purposes. Some lasers emit light at different wavelengths under different conditions. For example, the heliumneon laser is best known for its red output at 632.8 nm. However, the same gas mixture can be used with different optics to emit green light at 543 nm, or to emit invisible infrared light at wavelengths as long as 3393 nm.
Some lasers can emit two or more wavelengths at once, called multiline operation. In some cases, the wavelengths are close together. The argon laser emits several wavelengths between 450 and 530 nm, with the strongest lines at 514.5 and 488 nm. In others, they are widely separated, as in helium-neon. Some lasers emit many wavelengths in a limited range; one example is the carbon-dioxide laser, with dozens of wavelengths between 9 and 11 µm (9000 to 11,000 nm) in the infrared.
As we will see later, the way lasers emit light tends to concentrate energy at specific wavelengths. However, some lasers can emit light over a fairly wide range of wavelengths. The most important examples are lasers in which light is emitted by organic dyes in solution or certain solid-state crystals. They can be tuned in wavelength by adjusting the laser's optical system.
Output Power
Output power measures the strength of a laser beam, which differs widely among lasers. Strictly speaking, power measures how much energy the laser releases per unit time; it is measured in watts and defined by the formula:
Power = [DELTA](Energy)/[DELTA](Time)
One watt is defined as a rate of one joule (of energy) per second.
Laser output powers cover a wide range. Some lasers produce beams containing less than a thousandth of a watt (a milliwatt). Others produce thousands of watts (kilowatts).
(Continues...)
Excerpted from Understanding Lasers by Jeff Hecht Excerpted by permission.
All rights reserved. No part of this excerpt may be reproduced or reprinted without permission in writing from the publisher.
Excerpts are provided by Dial-A-Book Inc. solely for the personal use of visitors to this web site.
Table of Contents
Preface xiiiCHAPTER 1 Introduction and Overview 1
1.1 Lasers, Optics, and Photonics 1
1.2 Understanding the Laser 3
1.3 What Is a Laser? 4
1.4 Laser Materials and Types 8
1.5 Optical Properties of Laser Light 10
1.6 How Lasers Are Used? 14
1.7 What Have We Learned? 17
CHAPTER 2 Physical Basics 21
2.1 Electromagnetic Waves and Photons 21
2.2 Quantum and Classical Physics 29
2.3 Interactions of Light and Matter 39
2.4 Basic Optics and Simple Lenses 47
2.5 Fiber Optics 51
2.6 What Have We Learned? 54
CHAPTER 3 How Lasers Work 59
3.1 Building a Laser 59
3.2 Producing a Population Inversion 60
3.3 Resonant Cavities 66
3.4 Laser Beams and Resonance 73
3.5 Wavelength Selection and Tuning 81
3.6 Laser Excitation and Efficiency 85
3.7 What Have We Learned? 89
CHAPTER 4 Laser Characteristics 95
4.1 Coherence 95
4.2 Laser Wavelengths 98
4.3 Properties of Laser Beams 103
4.4 Laser Power 108
4.5 Laser Efficiency 110
4.6 Pulse Characteristics 115
4.7 Polarization 120
4.8 What Have We Learned? 121
CHAPTER 5 Optics, Laser Accessories, and Measurements 127
5.1 Classical Optical Devices 127
5.2 Optical Materials 136
5.3 Optical Coatings and Filters 141
5.4 Beam Delivery, Direction, and Propagation 145
5.5 Mounting and Positioning Equipment 148
5.6 Nonlinear Optics 149
5.7 Beam Modulation and Output Control 156
5.8 Measurements in Optics 159
5.9 What Have We Learned? 164
CHAPTER 6 Laser Types, Features, and Enhancements 169
6.1 Perspectives on Laser Types 169
6.2 Laser Media 171
6.3 Pumping and Energy Storage 177
6.4 Laser Pulse Characteristics 182
6.5 Wavelength Conversion 195
6.6 Laser Oscillators and Optical Amplifiers 201
6.7 Wavelength Options 207
6.8 Laser-Like Light Sources 209
6.9 What Have We Learned? 211
CHAPTER 7 Gas Lasers 217
7.1 The Gas-Laser Family 217
7.2 Gas-Laser Basics 218
7.3 Helium–Neon Lasers 225
7.4 Argon- and Krypton-Ion Lasers 229
7.5 Metal-Vapor Lasers 232
7.6 Carbon Dioxide Lasers 235
7.7 Excimer Lasers 240
7.8 Nitrogen Lasers 243
7.9 Chemical Lasers 243
7.10 Other Gas Lasers 246
7.11 What Have We Learned? 247
CHAPTER 8 Solid-State Lasers 253
8.1 What Is a Solid-State Laser? 253
8.2 Solid-State Laser Materials 258
8.3 Solid-State Laser Configurations 265
8.4 Major Solid-State Laser Materials 271
8.5 Optically Pumped Semiconductor Lasers 284
8.6 Broadband and Tunable Solid-State Lasers 288
8.7 Pulsed Solid-State Lasers 294
8.8 What Have We Learned? 295
CHAPTER 9 Fiber Lasers and Amplifiers 301
9.1 What Are Fiber Lasers? 301
9.2 Optical Fiber Structures 306
9.3 Fiber Laser Design and Efficiency 310
9.4 Rare-Earth-Doped Fiber Lasers 318
9.5 Rare-Earth-Doped Fiber Amplifiers 328
9.6 Raman Fiber Lasers and Amplifiers 332
9.7 What Have We Learned? 335
CHAPTER 10 Diode and Other Semiconductor Lasers 341
10.1 Types of Semiconductor Lasers 341
10.2 Development of Diode Lasers 342
10.3 Semiconductor Basics 344
10.4 Comparing LED and Diode-Laser Emission 353
10.5 Confining Light and Current 359
10.6 Edge-Emitting Diode Lasers 370
10.7 Surface-Emitting Diode Lasers 375
10.8 Optical Properties of Diode Lasers 379
10.9 Diode-Laser Materials and Wavelengths 381
10.10 Quantum Cascade Lasers and Related Types 390
10.11 What Have We Learned? 393
CHAPTER 11 Other Lasers and Laser-Like Sources 399
11.1 Tunable Dye Lasers 399
11.2 Optical Parametric Sources 404
11.3 Supercontinuum Sources 408
11.4 Frequency Combs 408
11.5 Extreme Ultraviolet Sources 410
11.6 Free-Electron Lasers 416
11.7 What Have We Learned? 420
CHAPTER 12 Low-Power Laser Applications 425
12.1 Advantages of Laser Light 426
12.2 Reading with Lasers 433
12.3 Optical Disks and Data Storage 437
12.4 Laser Printing 440
12.5 Lasers in Fiber-Optic Communications 442
12.6 Laser Measurement 447
12.7 Laser Light Shows, Pointers, and Projection Displays 453
12.8 Low-Power Defense Applications 456
12.9 Sensing and Spectroscopy 459
12.10 Holography 464
12.11 Other Low-Power Applications 468
12.12 What Have We Learned? 469
CHAPTER 13 High-Power Laser Applications 475
13.1 High- Versus Low-Power Laser Applications 475
13.2 Attractions of High-Power Lasers 476
13.3 Important Considerations and Trends 477
13.4 Materials Working 481
13.5 Additive Manufacturing and Three-Dimensional Printing 489
13.6 Semiconductor Electronics Fabrication 491
13.7 Laser Medical Treatment 493
13.8 Photochemistry and Isotope Separation 501
13.9 Laser-Driven Nuclear Fusion 503
13.10 High-Energy Laser Weapons 505
13.11 What Have We Learned? 510
CHAPTER 14 Lasers in Research 515
14.1 Lasers Open New Opportunities 515
14.2 Laser Spectroscopy 516
14.3 Manipulating Tiny Objects 521
14.4 Atom Lasers and Bose–Einstein Condensates 522
14.5 Detection of Gravitational Waves 524
14.6 Laser Guide Stars for Astronomy 525
14.7 Slow Light 526
14.8 Nanoscale Lasers 527
14.9 Strange Lasers 529
14.10 Extreme Power Ultrashort Pulse Lasers 530
14.11 X-Ray Free-Electron Lasers 535
14.12 Other Emerging Research 536
14.13 What Have We Learned? 538
Answers to Quiz Questions 543
Appendix A: Laser Safety 547
Appendix B: Handy Numbers and Formulas 553
Appendix C: Resources and Suggested Readings 557
Glossary 561
Index 575
What People are Saying About This
"This book is an easy-to-follow guide that requires a minimal background in algebra. The use of simple language, drawings, tables and multiple-choice quizzes make this book an ideal text for advanced high school students, undergraduates studying physics and engineering, and professionals who work with lasers but lack a formal knowledge of the subject." (Optics & Photonic News, April 2009)
"College-level libraries strong in science and technology titles will appreciate this easy introduction guide to laser technology, which moves from the foundations of how lasers work and how they are used to discussions of specific advanced laser types, applications, and the science involved." (The Midwest Book Review, September 2008)