Starting with the basic principles and components of the nervous system with sensory receptors, neurons and dendrites, and the skeletal muscle circuits the authors unfold the mystery of this communication with simple, elegant mathematical formulas to enhance your understanding of how the nervous system functions in complicated auditory and visual systems, and the brain.
Includes extensive references at the end of each chapter!"
Sponsored by:
IEEE Engineering in Medicine and Biology Society
Starting with the basic principles and components of the nervous system with sensory receptors, neurons and dendrites, and the skeletal muscle circuits the authors unfold the mystery of this communication with simple, elegant mathematical formulas to enhance your understanding of how the nervous system functions in complicated auditory and visual systems, and the brain.
Includes extensive references at the end of each chapter!"
Sponsored by:
IEEE Engineering in Medicine and Biology Society
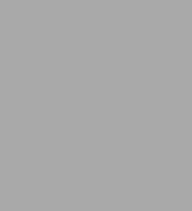
Understanding the Nervous System: An Engineering Perspective
412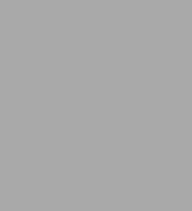
Understanding the Nervous System: An Engineering Perspective
412Paperback
-
SHIP THIS ITEMIn stock. Ships in 1-2 days.PICK UP IN STORE
Your local store may have stock of this item.
Available within 2 business hours
Related collections and offers
Overview
Starting with the basic principles and components of the nervous system with sensory receptors, neurons and dendrites, and the skeletal muscle circuits the authors unfold the mystery of this communication with simple, elegant mathematical formulas to enhance your understanding of how the nervous system functions in complicated auditory and visual systems, and the brain.
Includes extensive references at the end of each chapter!"
Sponsored by:
IEEE Engineering in Medicine and Biology Society
Product Details
ISBN-13: | 9780879422967 |
---|---|
Publisher: | Wiley |
Publication date: | 01/22/1993 |
Series: | IEEE Press Understanding Science & Technology Series , #8 |
Pages: | 412 |
Product dimensions: | 6.10(w) x 9.07(h) x 0.99(d) |
About the Author
Read an Excerpt
Understanding the Nervous System
By Sid Deutsch
John Wiley & Sons
ISBN: 0-8794-2296-3Chapter One
Excitable Tissue
ABSTRACT. The nervous system includes voluntary and autonomic (sympathetic and parasympathetic) systems. This book is devoted almost entirely to the voluntary system.
The system consists mostly of excitable tissue-sensory receptors, neuron cell bodies, axons, and muscle fibers. If you step on a sharp object, it stimulates sensory receptors that in turn stimulate neurons; the latter send action potentials (APs) via axons to interneurons and motoneurons in the spinal cord. The motoneurons send APs to the appropriate muscles, which contract so as to make you jump off the object.
Some of the activity involves atomic dimensions and, because distances are so small, time intervals are correspondingly small. It takes a factor of about [10.sup.7] to transform atomic distances into dimensions that are familiar to us. For example, most atoms and simple compounds, if magnified by [10.sup.7], turn out to be 3 mm (0.12 in.) in diameter. One centimeter multiplied by [10.sup.7] equals 100 km (62 mi.). In time, one second multiplied by [10.sup.7] is almost equal to 4 months.
Sensory receptors are usually at rest when they are unstimulated. Neuron cell bodies are at rest when they are not generating APs, while muscle fibers and axons are at rest when they are not carrying APs (that is, no APs are propagating along the muscle fiber or axon).
Body tissues are bathed in fluid that has an excess of sodium and chloride ions. Internally, excitable tissue at rest has an excess of potassium and large organic negative ions. This external-internal combination forms a battery that makes the inside of the tissue 60 to 90 mV more negative than the outside. The electric field across the membrane is very high-up to 12,000 V/mm. The physiologically compatible ionic concentrations are maintained by sodium and potassium pumps.
1-1 Introduction
This book is about the nervous system, about how its components function and communicate with each other. The book does not read like a novel. There are drawings, visual images to be stored away in memory along with associated interpretations. There are tables that have to be slowly digested so that your nervous system can store the meanings conveyed by the numbers and by the symbols we call the English and Greek alphabets. To some extent one must learn a new vocabulary, the language of the neurophysiologist. It is a difficult subject [D. Junge, 1981; J. Kline, 1976]. However, familiar words such as "voltage" and "potential" appear throughout because the messages of the nervous system, in addition to relatively slow-speed chemical signals, are transmitted as voltages (and their accompanying currents) [M. V. L. Bennett, 1977].
What are we trying to accomplish in this book? The nervous system from an engineering perspective is quite different from the nervous system as seen by a life scientist. The life scientist is trained to work with living tissue-with the nervous systems of insects, animals, and human patients. He or she painstakingly uncovers the chemical, electrical, and mechanical details behind the behaviors exhibited by these nervous systems. Papers and books written by life scientists are likely to contain page after page of descriptive material. Organic molecules, amino acids, and proteins are frequently mentioned. The engineer, on the other hand, is trained to set up block diagrams and mathematical models. To a biomedical engineer, a model is an approximate representation of a biological system. Most biological systems are nonlinear, so the engineer sometimes comes up with a linear system that is a gross approximation. We try to avoid, of course, models that are excessively inaccurate. We try to generate models that reasonably simplify the biological system while retaining some semblance of accuracy.
With further regard to models and accuracy: Our first encounter with a model, in this chapter, yields the calculated value ITLITL = 0.9444 µF/[cm.sup.2] for the capacitance of a membrane. The four-place accuracy is somewhat comical because, given uncertainties associated with the dielectric constant and membrane thickness, the real accuracy is probably ±20%. Physiologists use the value ITLITL = 1 µF/[cm.sup.2], and rightly so. For engineering students, however, in this book we give the equation's four-place answer because this allows the reader to check his or her work. The reader has to be reasonably sophisticated in judging the real accuracy of a four-place value. In the same equation, for example, the permittivity of a vacuum is given as 8.854 × [10.sup.-12] F/m; this is accurate to four places.
Biomedical and clinical engineers greatly contribute to improving the quality of human life by designing (and frequently operating) much of the equipment in a modern laboratory, and by designing prosthetic devices. They have to work with biologists and medical doctors. Unfortunately, engineers and life scientists represent two different cultures. Before they try to communicate with each other, each should learn some of the language of the other's discipline. It is hoped that this book will enable engineers to understand and appreciate some of the problems encountered by neurophysiologists.
Although insect as well as human nervous systems are mentioned earlier, the book actually concentrates on mammalian models. Most of the biomedical engineers who specialize in the nervous system naturally gravitate toward mammalian systems. One should mention, however, that many of the details of an actual neural network have been traced out in the snail Aplysia. That creature is almost transparent, thereby exposing its abdominal ganglia to the microelectrode probes of neurophysiologists. Still more remarkable, a voltage-sensitive dye can be applied so that electrical activity becomes visible. In a large population of Aplysia neurons, where it is only feasible to probe a few with microelectrodes, it is actually possible to see that hundreds are active during, say, the gill-withdrawal reflex [D. Zecevic etal., 1989].
From the chapter headings in this book, it is obvious that the reader is gradually led along various foundation paths until the climax, the brain, is reached in the last chapter (Chap. 12).
What about the relatively new field of artificial neural networks (ANNs)? Well, real neural networks are considered throughout the book, but especially in Chapter 11. It is reasonable to also devote a section to ANNs in Chapter 11 (Sect. 11-5; see also Sects. 7-3 and 7-4). First, it is "cost effective" because the same techniques, such as learning, self-organization, associative memory, and parallel processing, are common to real and artificial neural networks. Second, one can argue that many of the networks that are labeled "hypothetical" in this book should more properly be labeled "artificial." Third, people who invent ANNs are forever hopeful that these networks will turn out to be "real." Their publications have been rightly criticized for omitting the "artificial" designation, even when the network has obviously nothing in common with living structures. In short, some "neural networks" are real, some are artificial, and some possess aspects of both of these states.
When we speak of the nervous system we usually think of its glamorous components-those that are involved in the writing of books, music, and equations; those that are involved in the major-league launching of baseballs, tennis balls, and golf balls; and so forth. These activities are controlled by the voluntary or somatic nervous system. (Somatic here pertains to the framework of the body.) By contrast, there is also an involuntary, visceral, autonomic nervous system, which actually consists of two systems-the sympathetic and parasympathetic. [The viscera are the internal organs of the body, especially those contained within the abdominal (intestinal) and thoracic (chest) cavities. Autonomic refers to involuntary, spontaneous activity.] [A. C. Guyton, 1986; T. C. Ruch et al., 1965; T. C. Ruch and H. D. Patton, 1979]
The autonomic nervous system is devoted to "housekeeping" chores that we cannot consciously control. Glands, smooth muscles, and the heart are innervated by this system. A typical example, in Fig. 1-1, is provided by the heart's pacemaker, the internal mechanism that determines when the cardiac muscle should contract. The pacemaker is connected to both the sympathetic and parasympathetic systems. When the former is excited, it speeds up the pacemaker; when the parasympathetic fibers are excited, the pacemaker slows down. Each system is part of a feedback loop as shown in Fig. 1-1.
The dual control illustrated in Fig. 1-1 applies to most of the organs innervated by the autonomic system; that is, if the sympathetic system causes a muscle to contract (or relax), the parasympathetic system causes it to relax (or contract). Muscles controlled by the autonomic system are visceral smooth muscles; in contrast, those controlled by the voluntary system are skeletal striated muscles (fibers having transverse stripes). Cardiac and smooth muscles are similar in that they are both stimulated by the autonomic nervous system.
This book is devoted almost entirely to the voluntary, somatic nervous system. The heart is avoided because it is too specialized; entire books are dedicated to this organ alone, including biomedical engineering texts [W. Welkowitz, 1987]. Fortunately, much of the discussion of the voluntary nervous system is almost directly applicable also to the autonomic system.
Another area omitted in this book is that of the electromyogram (EMG). This is a record of the electric response that accompanies a muscle contraction. It forms an important niche in biomedical engineering but, like the heart, it is avoided because it is too specialized. The interested reader can start with the work of Carlo J. DeLuca and his colleagues [R. S. LeFever and C. J. DeLuca, 1982; F. B. Stulen and C. J. DeLuca, 1981]. However, typical feedback loop circuits of skeletal muscles are considered in Chapter 8 because the axons to and from muscles are part of the peripheral nervous system. (The brain and spinal cord form the central nervous system.)
At the outset it is appropriate to point out that this book does not regard extrasensory perception as a valid topic in a scientific discussion of the nervous system. Those who are interested in ectoplasm and the like should refer to the Skeptical Inquirer magazine (published by the Committee for the Scientific Investigation of Claims of the Paranormal).
[S. D. writes: "How did I, an electrical engineer, only mildly neurotic, get into this business? By way of a biomedical engineering program that some of my colleagues organized at the Polytechnic Institute of Brooklyn in the early 1960s. It seemed to me that much of the nervous system is reminiscent of an electrical communications network. I began to work with neurophysiologists, biologists, and medical doctors, and to read their literature. I gathered material for biomedical engineering courses, especially one on 'Neuroelectric Systems.' And so, like a tadpole turning into a frog, I metamorphosed into a biomedical engineer."]
1-2 Various Types of Excitable Tissue
Much of what we know about the nervous system has been donated by lower animals, such as the squid, to cite one example. This creature has a large nerve fiber or axon whose diameter is 0.5 mm (0.02 in.). After the axon is dissected out of the squid (and, incidentally, the animal's minuscule brain is no longer transmitting pain messages along its oversized axon) we can insert a fine wire electrode into the axon and make all kinds of measurements. Besides teaching us a great deal about how the nervous system functions, the experiments reveal that the nervous system of a squid is similar in many respects to that of a human, despite the huge genetic distance between the two species, because of the unified way in which evolution has progressed from animal to animal.
The nervous system consists mostly of excitable tissue-tissue that responds to a stimulus. As Luigi Galvani (1737-1798) and Alessandro Volta (1745-1827) discovered, if you suitably apply voltage to the leg of a frog it will jump (if the leg is alive, of course). The muscle is excitable tissue.
It is easy enough to find nonexcitable tissue. Consider a beautiful coleus plant, for example: if the wind blows, it responds by bending over, but that is not what we mean by the "response to a stimulus." Or take a piece of skin that is being grafted from one part of the body to another-it is living tissue, unlike a Band-Aid, but it does not respond to a voltage or any other conventional stimulus.
To be more specific, there are four kinds of excitable tissue: sensory receptors, neuron cell bodies, axons, and muscle fibers. (The branches feeding into a neuron cell body, called dendrites, are very much a part of the nervous system, but are omitted from this list because they are passive and unexcitable.) All of these tissues are called into play when you step on a mildly noxious stimulus such as a sharp pebble. Here the sensory receptors are pressure and pain transducers; they respond by generating a voltage called a receptor potential. Sensory receptors are usually associated with a neuron, a cell that is specialized in that it, in turn, responds to the stimulus by generating a rapid burst of voltage pulses. (Here a voltage pulse is a relatively high voltage that lasts for a relatively short period of time. A somewhat different type of neuron, which generates a smoothly varying voltage, or graded potential, is discussed in subsequent chapters.) [C. F. Stevens, 1979]
The situation is more clearly depicted in Fig. 1-2. The stimulus-in this case, a constant high pressure that results in excessive stress or damage-is accompanied by a pain receptor potential. At first the potential is relatively high, but having "sounded the alarm," it soon decreases to a much lower, steady level. This decrease is called adaptation. The neuronal output corresponding to the receptor potential, the 0.1-V high "spikes," are called action potentials (APs). If you could hear the neuronal response, it would sound like machine-gun fire; in fact, physiologists frequently amplify the neuronal output and listen to the rat-a-tat-tat while their eyes are occupied in adjusting the electrodes.
The magnitude of the APs, 0.1 V, has been the same in every animal from before the squid to whatever comes after man, a period of some 500 million years thus far.
Today, voltage labels are familiar to everyone. A cell in the ubiquitous small chemical battery supplies 1.5 V; automobile batteries generate 12 V; home equipment operates on either 110 or 220 V, depending on the country; and so forth. Unfortunately, the early neurophysiologists preferred the word "potential" to "voltage." If you ask for a "1.5-potential battery," you will get an incredulous "What?" even at a Radio Shack store. In this book, however, we will side with the neurophysiologists: a sensory receptor generates receptor potentials, and a neuron generates action or graded potentials.
One part of the body also communicates with another part via chemical messengers, but these are relatively slow. To jump off that noxious pebble you need a high-speed channel. The neuron sends its AP burst along a nerve axon to the base of the spinal cord, as illustrated in Fig. 1-3 (any resemblance here to persons living or dead is purely coincidental). The axon leading to the spinal cord is afferent; that is, directed from the periphery of the body inward to the spinal cord or brain. Propagation along an axon is analogous to the transmission of electrical signals along a copper wire. The axon is quite remarkable in that it is an unbroken length of tissue, like a very fine wire, some three feet long for a fiber that runs from the foot of an adult to his or her spinal cord. The particular axon that carries the high-speed "Ouch" message has a diameter of 0.02 mm (0.0008 in.), and the velocity of signal propagation is 120 m/s (400 ft/s or 270 mph).
(Continues...)
Excerpted from Understanding the Nervous System by Sid Deutsch Excerpted by permission.
All rights reserved. No part of this excerpt may be reproduced or reprinted without permission in writing from the publisher.
Excerpts are provided by Dial-A-Book Inc. solely for the personal use of visitors to this web site.
Table of Contents
Preface.Excitable Tissue.
Sensory Receptors.
Generation of the Action Potential.
Propagation of the Action Potential.
Dendritic Summation.
Lateral Inhibition.
Simple Neuronal Systems.
Skeletal Muscle Circuits.
The Auditory System.
The Eye as a Transducer.
Visual Pattern Recognition, Neural Networks, and "Household Chores".
About the Brain.
Index.