With over 90,000 workers in the water environment industry and technological developments rapidly advancing understanding in this area, water has become one of the most important issues of our time. Career scope for those working in the water and wastewater engineering sphere is expanding. This accessible student textbook introduces the reader to the key concepts of water technology by explaining the fundamentals of hydrobiology, aquatic ecosystems, water treatment and supply and wastewater treatment. The Water Framework Directive is the driving force in European water management and protection. Professor Gray uses the Water Framework Directive as the unifying theme of this new edition. This text provides a complete introduction to all aspects of managing the hydrological cycle and is ideal for those interested in a career in the water industry.For masters' students in evironmental science, engineering and construction courses and those in the CIWEM diploma, Water Technology, 3e, is an essential resource that will continue to be useful in professional life.
With over 90,000 workers in the water environment industry and technological developments rapidly advancing understanding in this area, water has become one of the most important issues of our time. Career scope for those working in the water and wastewater engineering sphere is expanding. This accessible student textbook introduces the reader to the key concepts of water technology by explaining the fundamentals of hydrobiology, aquatic ecosystems, water treatment and supply and wastewater treatment. The Water Framework Directive is the driving force in European water management and protection. Professor Gray uses the Water Framework Directive as the unifying theme of this new edition. This text provides a complete introduction to all aspects of managing the hydrological cycle and is ideal for those interested in a career in the water industry.For masters' students in evironmental science, engineering and construction courses and those in the CIWEM diploma, Water Technology, 3e, is an essential resource that will continue to be useful in professional life.
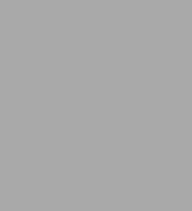
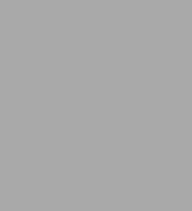
eBookRevised ed. (Revised ed.)
Available on Compatible NOOK devices, the free NOOK App and in My Digital Library.
Related collections and offers
Overview
With over 90,000 workers in the water environment industry and technological developments rapidly advancing understanding in this area, water has become one of the most important issues of our time. Career scope for those working in the water and wastewater engineering sphere is expanding. This accessible student textbook introduces the reader to the key concepts of water technology by explaining the fundamentals of hydrobiology, aquatic ecosystems, water treatment and supply and wastewater treatment. The Water Framework Directive is the driving force in European water management and protection. Professor Gray uses the Water Framework Directive as the unifying theme of this new edition. This text provides a complete introduction to all aspects of managing the hydrological cycle and is ideal for those interested in a career in the water industry.For masters' students in evironmental science, engineering and construction courses and those in the CIWEM diploma, Water Technology, 3e, is an essential resource that will continue to be useful in professional life.
Product Details
ISBN-13: | 9780080959641 |
---|---|
Publisher: | CRC Press |
Publication date: | 10/28/2010 |
Sold by: | Barnes & Noble |
Format: | eBook |
Pages: | 746 |
File size: | 13 MB |
Note: | This product may take a few minutes to download. |
Read an Excerpt
Water Technology
An Introduction for Environmental Scientists and EngineersBy N. F. Gray
Butterworth-Heinemann
Copyright © 2010 Professor N.F. GrayAll right reserved.
ISBN: 978-0-08-095964-1
Chapter One
Basic Considerations in Hydrobiology
1.1 HYDROLOGICAL CYCLE
Water supplies are not pure in the sense that they are devoid of all dissolved chemical compounds like distilled–deionized water, but are contaminated by a wide range of trace elements and compounds. In the early days of chemistry, water was known as the universal solvent, due to its ability to slowly dissolve into solution anything it comes into contact with, from gases to rocks. So as rain falls through the atmosphere, flows over and through the Earth's surface, it is constantly dissolving material, forming a chemical record of its passage from the clouds. Therefore, water supplies have a natural variety in quality, which depends largely on their source.
The total volume of water in the world remains constant. What changes is its quality and availability. Water is constantly being recycled, a system known as the water or hydrological cycle. Hydrologists study the chemical and physical nature of water, and its movement both on and below the ground. In terms of total volume, 97.5% of the world's water is saline with 99.99% of this found in the oceans, and the remainder making up the salt lakes. This means that only 2.5% of the volume of water in the world is actually non-saline. However, not all of this freshwater is readily available for use by humans. Some 75% of this freshwater is locked up as ice caps and glaciers, although this is slowly changing in response to climate change, with a further 24% located under ground as groundwater, which means that less than 1% of the total freshwater is found in lakes, rivers and the soil (Table 1.1). Only 0.01% of the world's water budget is present in lakes and rivers, with another 0.01% present as soil moisture but unavailable to humans for supply. So while there appear to be lots of water about, there is in reality very little which is readily available for use by humans. Within the hydrological cycle, the water is constantly moving, driven by solar energy. The sun causes evaporation from the oceans, which forms clouds and precipitation (rainfall). Evaporation also occurs from lakes, rivers and the soil; with plants contributing significant quantities of water by evapotranspiration. While about 80% of precipitation falls back into the oceans, the remainder falls onto land. It is this water that replenishes the soil and groundwater, feeds the streams and lakes, and provides all the water needed by plants, animals and, of course, humans (Fig. 1.1). The cycle is a continuous one and so water is a renewable resource (Franks, 1987). In essence, the more it rains, the greater the flow in the rivers and the higher the water table rises as the underground storage areas (i.e. the aquifers) fill with water as it percolates downwards into the earth. Water supplies depend on rain, so when the amount of rain decreases, the volume of water available for supply will decrease, and in cases of severe drought will fall to nothing. Therefore, in order to provide sufficient water for supply all year round, careful management of resources is required.
Nearly all freshwater supplies come from precipitation which falls onto a catchment area. Also known as a watershed or river basin, the catchment is the area of land, often bounded by mountains, from which any water that falls into it will drain into a particular river system. A major river catchment will be made up of many smaller sub-catchments each draining into a tributary of the major river. Each sub-catchment will have different rock and soil types, and each will have different land use activities which also affect water quality. So the water draining from each sub-catchment may be different in terms of chemical quality. As the tributaries enter the main river they mix with water from other sub-catchments upstream, constantly altering the chemical composition of the water. Therefore, water from different areas will have a unique chemical composition.
When precipitation falls into a catchment one of three major fates befalls it:
1. It may remain on the ground as surface moisture and be eventually returned to the atmosphere by evaporation. Alternatively, it may be stored as snow on the surface until the temperature rises sufficiently to melt it. Storage as snow is an important source of drinking water in some regions. For example, throughout Scandinavia lagoons are constructed to collect the run-off from snow as it melts, and this provides the bulk of the drinking water during the summer.
2. Precipitation flows over the surface into small channels becoming surface run-off into streams and lakes (Section 2.1). This is the basis of all surface water supplies with the water eventually evaporating into the atmosphere, percolating into the soil to become groundwater, or continuing as surface flow in rivers back to the sea.
3. The third route is for precipitation to infiltrate the soil and slowly percolate into the ground to become groundwater that is stored in porous sediments and rocks. Groundwater may remain in these porous layers for periods ranging from just a few days to possibly millions of years (Section 2.2). Eventually groundwater is removed by natural upward capillary movement to the soil surface, plant uptake, groundwater seepage into surface rivers, lakes or directly to the sea, or artificially by pumping from wells and boreholes.
Therefore, from a management perspective the hydrological cycle at this point can be represented as:
P = E + R + I (1.1)
where P is the precipitation, E is the evapotranspiration, R is the run-off and I is the infiltration. In France, approximately 55% of precipitation evaporates, 25% becomes surface run-off and 20% infiltrates into the ground. However, this will vary enormously between areas.
The water in the oceans, ice caps and aquifers is all ancient, acting as sinks for pollutants. All pollution eventually ends up in the cycle and will ultimately find its way to one of these sinks. Freshwater bodies are often closely interconnected, forming part of a continuum from rainwater to saline (marine) water, and so may influence each other quite significantly.
In many countries the hydrological cycle is highly managed in order to provide sufficient water for industry, agriculture and domestic use. This requires the management of surface and groundwater resources, the treatment and supply of water, its subsequent collection, cleansing and return to the cycle (Fig. 1.2). The hydrological cycle will be increasingly affected by climate change through rising surface temperatures increasing the rates of precipitation, evaporation and evapotranspiration. This will result in some regions becoming wetter and some subtropical areas in particular becoming even drier, increasing the incidence of droughts. At higher latitudes changes in snowfall patterns may lead to springtime flooding with the proportion of water stored in the cycle as ice slowly declining with sea levels rising to accommodate the extra free water (Section 1.5).
1.2 Comparison Between Freshwater and Saline Water
Conditions in freshwater differ from saline water in many ways; for example, lower concentrations of salts (especially Na, SO4, Cl) and smaller volumes of water. Smaller volumes result in a greater range and more rapid fluctuations in temperature, oxygen and other important parameters, making freshwater ecosystems more vulnerable to pollution. The smaller volume and area of freshwater bodies also acts as a physical restriction on certain species due to size and feeding behaviour, restricting free dispersion of organisms. Rivers and lakes are isolated entities that make colonization difficult, resulting in comparatively low diversities. In terms of pollution, both lakes and oceans act as sinks, with the former having only limited dilution and assimilative capacity.
Freshwaters have a low concentration of salts, which presents organisms living in such waters with two problems: first, how to acquire and retain sufficient minimum salts for metabolism; and, second, how to maintain concentrations of salts in their internal fluids that are higher than those in the water (i.e. osmotic and diffusion problems). To some extent this is why the total number of species of organisms that live in freshwater is smaller than the number living wholly on land or in the sea.
1.3 Freshwater Habitats
There are a wide range of freshwater habitats. For example, there are lakes, ponds, turrlochs (temporary ponds), springs, mountain torrents, chalk streams, lowland rivers, large tropical lakes, and a range of artificial habitats such as abandoned peat workings (e.g. the Norfolk Broads) and gravel pits. These are just a few of the wide variety of freshwater bodies that can be found and the hydrological, physico-chemical and biological characteristics vary enormously between them.
In the most basic classification water can be separated into surface and groundwater, with surface waters further split into flowing systems (lotic) and standing systems (lentic). In reality, lotic and lentic systems often grade into each other and may be difficult in practice to differentiate. Lakes generally have an input and an output with what may be a very small throughput. Therefore, these systems can only be separated by considering the relative retention times (Table 1.2). For example, many rivers have been impounded along their length to form reservoirs resulting in lentic–lotic (e.g. River Severn in England) or lotic–lentic–lotic systems (e.g. River Liffey in Ireland).
Rivers can be hydraulically defined as having a unidirectional flow, with generally short retention times measured in days rather than weeks. They have a relatively high average flow velocity of between 0.1 and 1.0ms-1, although the discharge rate (m3s-1) can be highly variable over time, and is a function of climatic and discharge conditions within the watershed. Rivers generally display continuous vertical mixing due to currents and turbulence, although lateral mixing may only occur over considerable distances. Lakes in contrast have a low surface flow velocity of between 0.001 and 0.01ms-1, with water and element retention times varying from 1 month to > 100 years. Currents are multi-directional with diffusion an important factor in dispersion of pollutants (Section 9.2.2). Depending on climatic conditions and depth, lakes can display alternating periods of stratification and vertical mixing (Section 2.1.1). Groundwaters display a steady flow pattern in terms of direction and velocity, resulting in poor mixing. Average flow velocities are very low, between 10-10 and 10-3 ms-1. This is governed by the porosity and permeability of the aquifer material but results in very long retention periods (Table 1.2). There are a number of transitional forms of water bodies that do fit into the above categories. The most common are flood plains which are seasonal and intermediate between rivers and lakes; reservoirs which are also seasonal depending on water release or use and are intermediate between rivers and lakes; marshes which are intermediate between lakes and aquifers; while karst and alluvial aquifers are intermediate between rivers and groundwater because of their relatively rapid flow. The Water Framework Directive recognizes not only surface freshwater and groundwater resources, but also transitional waters such as wetlands, estuaries and coastal waters that are highly influenced by freshwater inputs, in the overall management approach of catchment areas (Section 7.3).
The hydrodynamic characteristics of a water body are dependent primarily on the size of the water body and the climatic and drainage conditions within the catchment area. More specifically, rivers are further characterized by their discharge variability, lakes by their retention time and thermal regime controlling stratification, and groundwaters by their recharge regime (i.e. infiltration through the unsaturated aquifer zone to replenish the water stored in the saturated zone).
Lotic systems have a more open system than lentic ones, with a continuous and rapid throughput of water and nutrients, resulting in a unique flora and fauna (biota). The main advantages of aquatic habitats, and of lotic systems in particular, are that there is no gravity; food comes to the consumer; and that there are no waste disposal problems for the biota (Chapter 4).
1.4 The Catchment as Area Of Study
Water bodies and lotic systems in particular are not isolated entities; they impinge on the atmosphere and the land. Across these boundaries there is a constant movement of materials and energy. Across the air–water interface gases, water, dissolved nutrients and particles in rain all enter the river. The land–water interface is the main source of material from weathered and eroded rocks, and from dead and decaying plants and animals. Other factors such as transpiration rate of trees, soil and rock type, and land use all affect water quality, therefore it is necessary to study and manage the catchment area or watershed as a whole. This facilitates integrated management of the water cycle within that area.
Rivers have been managed on a catchment basis for many years although it is relatively recently, with the introduction of geographical information system (GIS) and remote sensing, that it has been possible to integrate land use and groundwater protection in water quality management at the catchment level (Chapter 7). However, groundwater resources do not always correspond to river catchment areas and often overlap catchment boundaries. Catchment management in Europe is currently being harmonized through the introduction of the EU Directive Establishing a Framework for Community Action in the Field of Water Policy (2000/60/EC). The Water Framework Directive (WFD), as it is generally known, proposes a new system of water quality management based on natural river catchments. It provides an integrated approach to the protection, improvement and sustainable use of European water bodies, including lakes, rivers, estuaries, coastal waters and groundwaters. Water resources are utilized for a wide range of uses including abstraction, fisheries, conservation, navigation, recreation and waste disposal. The WFD allows all these users (i.e. stakeholders) to have an active role in the management of their water resources. Under this legislation catchments are split into River Basin Districts (RBDs) and then managed through River Basin Management Plans (RBMPs). In Ireland, catchments have been combined to form eight RBDs of which three are International RBDs (i.e. transboundary) (Fig. 1.3). The Water Framework Directive aims to prevent further deterioration of water quality of all water resources and raise their minimum chemical and ecological status to a newly defined good quality status by 2012. Also by eliminating the discharge of certain hazardous substances to inland and groundwaters, it is expected that the concentrations of these pollutants in marine waters will eventually fall to background or zero levels. The Water Framework Directive is fully explored in Section 7.3.
1.5 Effects of Climate Change on Freshwaters
It is difficult to predict the effect climate change will have on freshwater ecosystems. Comparing the average temperature and total rainfall in England and Wales over the past 160 years clear changes have become discernible over the past three decades. By plotting the deviation of the summer and winter average temperature and total rainfall from 1845 to 1974 it can be seen that significant trends have evolved. On the summer chart recent years tend to fall more in the bottom right quarter, corresponding to hotter, drier summers compared with earlier periods (Fig. 1.4); while on the winter chart recent years fall more in the top right quarter, corresponding to warmer, wetter winters. However, it is very difficult to predict exactly how climate change will affect local and regional weather in either the short or medium to long term.
The ecological impact of climate change depends on the hydrological response of the local hydrological cycle at catchment level. The size of the catchment is very important, with smaller catchments most vulnerable to changes. Key effects include (i) increased water temperature; (ii) reduction in summer flow rates; (iii) rivers fed by groundwaters and upland peatlands possibly drying up in summer; and (iv) increased spates and flooding in winter. All surface waters are more vulnerable to acidification and eutrophication with reduced dilution increasing the impact of wastes generally. Lakes are especially vulnerable to climate change owing to a reduction in volume due to reduced inflow and surface run-off, and increased evaporation.
(Continues...)
Excerpted from Water Technology by N. F. Gray Copyright © 2010 by Professor N.F. Gray. Excerpted by permission of Butterworth-Heinemann. All rights reserved. No part of this excerpt may be reproduced or reprinted without permission in writing from the publisher.
Excerpts are provided by Dial-A-Book Inc. solely for the personal use of visitors to this web site.
Table of Contents
Basic Considerations in Hydrobiology; Water Resources and Demand; Factors Determining the Distribution of Animals and Plants in Freshwaters; Basic Aquatic Ecosystems; Micro-organisms and Pollution Control; Water Pollution; Water Basin Management; Water Quality and Regulation; Water Quality Assessment; Water Treatment and Distribution; Drinking Water Contamination; Pathogens and Their Removal; Nature of Wastewater; Introduction to Wastewater Treatment; Biological Aspects of Secondary Sewage Treatment; Fixed-film Systems; Activated Sludge; Other Biological Treatment Systems; Anaerobic Treatment; Physio-chemical Treatment Processes; Sludge Treatment and Disposal; Household and Small-scale Treatment Systems; Sustainability Principles in Water Management.