"An excellent introduction to complicated but fascinating subject." — Booklist.
This compelling book offers readers with no technical expertise beyond arithmetic an enlightening tour of the paradoxes inherent in the special theory of relativity, guided by a pair of eminent theoretical physicists.
Novel Prize physicist L. D. Landau and his distinguished colleague G. B. Rumer, employ a simple and straightforward manner to illuminate relativity theory's more subtle and elusive aspects. Using such familiar objects as trains, rulers, and clocks, the authors explain the reasoning behind seemingly self-contradictory ideas in which the relative seems absolute, but the absolute proves to be relative. A series of playful cartoons highlights the authors' witty observations on the laws governing inertia, the speed of light, the relationship of work and mass, and other relativistic concepts.
"The exposition is masterful . . . a superb book." — New York Times Book Review.
"An excellent introduction to complicated but fascinating subject." — Booklist.
This compelling book offers readers with no technical expertise beyond arithmetic an enlightening tour of the paradoxes inherent in the special theory of relativity, guided by a pair of eminent theoretical physicists.
Novel Prize physicist L. D. Landau and his distinguished colleague G. B. Rumer, employ a simple and straightforward manner to illuminate relativity theory's more subtle and elusive aspects. Using such familiar objects as trains, rulers, and clocks, the authors explain the reasoning behind seemingly self-contradictory ideas in which the relative seems absolute, but the absolute proves to be relative. A series of playful cartoons highlights the authors' witty observations on the laws governing inertia, the speed of light, the relationship of work and mass, and other relativistic concepts.
"The exposition is masterful . . . a superb book." — New York Times Book Review.
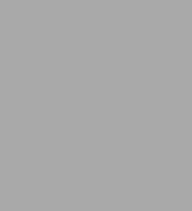
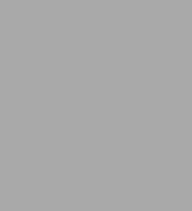
eBook
Available on Compatible NOOK devices, the free NOOK App and in My Digital Library.
Related collections and offers
Overview
"An excellent introduction to complicated but fascinating subject." — Booklist.
This compelling book offers readers with no technical expertise beyond arithmetic an enlightening tour of the paradoxes inherent in the special theory of relativity, guided by a pair of eminent theoretical physicists.
Novel Prize physicist L. D. Landau and his distinguished colleague G. B. Rumer, employ a simple and straightforward manner to illuminate relativity theory's more subtle and elusive aspects. Using such familiar objects as trains, rulers, and clocks, the authors explain the reasoning behind seemingly self-contradictory ideas in which the relative seems absolute, but the absolute proves to be relative. A series of playful cartoons highlights the authors' witty observations on the laws governing inertia, the speed of light, the relationship of work and mass, and other relativistic concepts.
"The exposition is masterful . . . a superb book." — New York Times Book Review.
Product Details
ISBN-13: | 9780486163482 |
---|---|
Publisher: | Dover Publications |
Publication date: | 12/26/2012 |
Sold by: | Barnes & Noble |
Format: | eBook |
Pages: | 80 |
File size: | 2 MB |
Read an Excerpt
What is Relativity?
By L. D. Landau, G. B. Rumer, N. Kemmer
Dover Publications, Inc.
Copyright © 2003 Dover Publications, Inc.All rights reserved.
ISBN: 978-0-486-16348-2
CHAPTER 1
Familiar Examples of Relativity
Does Every Statement Have a Meaning?
Evidently not. Even if you choose perfectly sensible words and put them together according to all the rules of grammar, you may still get complete nonsense. For instance the statement "This water is triangular" can hardly be given any meaning.
Unfortunately, however, not all examples of nonsense are so obvious and it often happens that a statement appears perfectly sensible at first sight but proves to be absurd on closer examination.
Right and Left
Look at the drawing on the next page. On which side of the road is the house—on the right or on the left? It is impossible to answer this question directly.
If you are walking from the bridge to the wood, the house will be on the left-hand side, but if you go from the wood to the bridge, you find the house on the right. Clearly, to speak of the right- or left-hand side of the road you must take into account the direction relative to which right or left is indicated.
It does make sense to speak of the right bank of a river, but only because the current determines the direction of the river. Likewise we can only say that cars keep to the right because the movement of a car singles out one of the two possible directions along the road.
We see that the notions "right" and "left" are relative: They acquire meaning only after the direction relative to which they are defined has been indicated.
Is It Day or Night Just Now?
The answer depends on where the question is being asked. When it is daytime in Moscow it is night in Vladivostok. There is no contradiction in this. The simple fact is that day and night are relative notions and our question cannot be answered without indicating the point on the globe relative to which the question is being asked.
Who Is Bigger?
In the first drawing on the opposite page, the shepherd is obviously bigger than the cow; in the second the cow is bigger than the shepherd. Again there is no contradiction. The reason is that the two drawings have been made by people observing from different points: One of them stood closer to the cow, the other closer to the shepherd. The picture is determined not by the actual sizes of the objects but by the angles at which they are seen. Evidently such angular dimensions of objects are relative. It makes no sense to speak of the angular dimensions of objects without indicating the point in space from which they are observed. For instance, to say, "This tower is seen under an angle of 45°," is to say precisely nothing. But the statement that the tower is seen under an angle of 45° from a point 15 meters (about 50 feet) away has a definite meaning; from this you can conclude that the tower is 15 meters high.
The Relative Seems Absolute
If the point of observation is moved a small distance, angular dimensions also change only by a small amount. That is why angular distances are often used in astronomy. A star map usually gives the angular distance between stars, i.e., the angle at which the distance between the two stars is observed from the surface of the earth.
We know that however much we move about on the Earth and whatever points on the globe we choose to observe from, we always see the stars in the sky at the same distances from each other. This is because the stars are such unimaginably large distances away from us that, in comparison, our movements on the Earth are negligible and can safely be forgotten. In this particular case we can therefore use angular distances as absolute measures of distance.
If we make use of the Earth's motion around the sun, it becomes possible to observe changes in angular distances between stars even though these changes are very small. But if we were to move our point of observation to some other star, such as Sirius, all angular distances would change so much that stars far apart in our sky might then be close together, and vice versa.
The Absolute Proves To Be Relative
We often use the words "up" and "down." Are these notions absolute or relative?
At different times in history the answers given to this question have differed. As long as people didn't know that the Earth is a sphere and thought that it was flat, like a pancake, the vertical was taken to be an absolute direction. It was taken for granted that this vertical direction was the same at all points on the Earth's surface, making it perfectly natural to speak of an absolute "up" and an absolute "down."
When it was proved that the Earth is a sphere the vertical began to totter—in people's minds. For if the Earth is a sphere, the direction of the vertical depends decisively on where on the Earth's surface the vertical is drawn.
Different points on the Earth will have different verticals. The notions "up" and "down" now cease to have meaning unless the point on the Earth's surface to which they refer is defined. Thus these notions change from absolute to relative ones. There is no unique vertical direction in the universe. Therefore, given any direction in space, we can find a point on the Earth's surface at which this direction is the vertical.
"Common Sense" Tries To Protest
Nowadays all this seems obvious and indisputable to us. But the record of history shows that in the past it was not so easy for mankind to understand the relativity of up and down. People have the tendency to ascribe absolute meaning to notions as long as their relative nature is not evident from everyday experience (as in the case of "right" and "left").
Remember the ridiculous objection to a spherical Earth which has come down to us from the Middle Ages: How could people possibly walk about upside-down?
The flaw in the reasoning here is that of not recognizing that, since the Earth is a sphere, the vertical is relative.
If you refuse to accept the principle of relativity for the vertical direction and assume, say, that the direction of the vertical in Moscow is absolute, you are bound to admit that the inhabitants of New Zealand walk upside-down. But if you do this, you should remember that for New Zealanders, the people in Moscow are the ones walking upside-down. This is no contradiction, because the notion of the vertical is not absolute but relative.
We should make a note of the fact that we begin to sense the real significance of the relativity of the vertical only when we consider two sufficiently distant parts of the Earth's surface, such as Moscow (or New York) and New Zealand. If we are concerned with two neighboring spots such as two houses in Moscow, we can for practical purposes take all verticals to be parallel, which means taking the vertical direction there to be absolute.
Only when we are obliged to deal with regions of a size comparable with the whole surface of the Earth do we find that trying to make use of an absolute vertical leads to absurdities and contradictions.
Our examples have shown that many of the notions in everyday use are relative, which means that they acquire meaning only when the conditions of observation are stated.
CHAPTER 2Space Is Relative
The Same Place or Not?
We often say that this event and that happened at the same place, and we are so used to saying this sort of thing that we tend to ascribe absolute meaning to such a statement. In truth it has no meaning at all! It is no better than saying "It is now five o'clock," without indicating whether it is supposed to be five o'clock in Moscow or in Chicago.
To show that this is so, let us imagine that two lady passengers traveling in the express from Moscow to Vladivostok have agreed to meet every day during the journey at the same place in the train, in order to write letters to their husbands. Their husbands will hardly agree that their wives have been meeting each day at one and the same place in space. On the contrary they will have every reason to state that the places at which their wives met from day to day were hundreds of miles apart. They received letters from Yaroslavl and from Perm, from Sverdlovsk and from Tyumen, from Omsk and from Khabarovsk.
So the two events—writing letters on the first and the second day of the journey—occurred at one and the same place from the point of view of the traveling ladies, but were many hundreds of miles apart from the point of view of their husbands.
Who is right, the travelers or their husbands? We have no reason to give preference to either. Quite clearly the notion "at the same place in space" has only relative meaning.
In the same way, the statement that two stars in the sky coincide has meaning only if we indicate that the observation is made from the Earth. We can speak of two events coinciding in space only if we indicate some objects relative to which the position of the events is determined.
Thus the notion of position in space is again a relative one. When we speak of the position of an object in space, we always assume this to mean its position relative to other objects. We have to admit that it is meaningless to demand that the whereabouts of one object be fixed without reference to other objects.
How Does a Body Move in Reality?
It follows from all this that the notion of "displacement of a body in space" also is relative. If we say that an object was displaced, this means no more than that it changed its position relative to other objects.
If the movement of an object is observed from different laboratories which are in motion relative to each other, the movement of the body will appear quite different.
A stone is dropped from a flying plane. Relative to the plane, the stone falls in a straight line; relative to the earth it describes a curve called a parabola.
But how does the stone move in reality?
This question has as little meaning as the question: "At what angle does one see the moon in reality?" Do you mean the angle at which it is seen from the sun or from the Earth?
The geometric form of a curve described by an object in motion is of the same relative nature as the photograph of a building. Depending on whether a house is photographed from the front or the back, different views are obtained. In the same way, depending on whether we observe the movement of an object from one laboratory or from another, we obtain different shapes of curve to describe its motion.
Are All Points of View Equivalent?
If in observing the motion of an object in space we were only interested in studying the shape of its trajectory (as the curve along which it moves is called), we would approach the question of choosing our place of observation with an eye to the convenience and simplicity of the resulting picture.
A good photographer choosing a place for his exposure is concerned with composition as well as with the beauty of the intended photograph.
However, when we study the displacement of objects in space we are interested in rather more. We do not want to know just the trajectory. We also want to be able to predict the trajectory along which the object will move under given conditions. In other words, we want to know the laws governing motion, the laws that force a body to move in just this way and no other.
Let us examine the question of the relativity of motion from this point of view; we shall see that not all situations in space are equivalent.
If you go to a photographer for a passport photograph, you naturally want him to take a photograph of your face, not of the back of your head. This demand determines the point in space from which the photographer must take the picture. We would say that any other position does not satisfy the condition imposed.
Rest Is Found!
The motion of objects is influenced by external actions. We call these actions forces. By studying the result of these actions we find that a completely new approach to the question of motion becomes possible.
Let us assume that we have available an object which is not acted on by any forces. We may position ourselves in different ways to observe it and accordingly will see it moving in different, more or less extraordinary ways. But it is impossible to deny that the most natural position for the observer will be the one from which the body appears to be simply at rest.
In this way we can give a completely new definition of rest, which does not depend on the displacement of the object in question relative to other objects. This is how we do it: An object on which no external forces are acting is in a state of rest.
The Laboratory at Rest
How can we bring this state of rest into being? When can we be sure that no forces whatsoever are acting on an object? Evidently we should remove the object as far as possible from all other objects that might act on it.
From such objects at rest we could at least in imagination construct a whole laboratory, and we could then speak of the properties of motion observed from this laboratory, which we call a laboratory at rest.
If the properties of a motion observed from any other laboratory differ from the properties of motion in the laboratory at rest, we have every right to assert that the first laboratory is moving.
Is the Train Moving?
Having established that in moving laboratories motion takes place according to other laws than in laboratories at rest, it would seem that the concept of motion loses its relative character. When henceforth we talk of motion, we may simply take this to mean motion relative to rest, and call such motion absolute.
But will any displacement of a laboratory lead us to observe that the laws of motion in it differ from those in a laboratory at rest?
We are sitting in a train running at constant speed along a straight track. We start observing the motion of objects in the railway carriage and comparing them with what takes place in a train at rest.
Everyday experience tells us that in such a train, moving in a straight line and at uniform speed, we do not notice any differences, compared to the motions of objects observed in a stationary train. If a ball is thrown up vertically inside a railway carriage, the ball will fall back into one's hands. A falling object will not describe a curve similar to the one drawn on the next page.
Apart from the shakes and bumps that are inevitable in practical running conditions, things take place in a uniformly moving railway carriage exactly as in a carriage at rest.
It is different if the carriage accelerates or slows down. In the former case we experience a jerk backward, in the latter, forward; in both we distinctly notice the difference compared to rest.
If the railway carriage continues to move steadily but with changing direction, we shall also sense it: At sharp right-hand curves we get thrown to the left; at left-hand turns, to the right.
Generalizing these observations, we reach this conclusion: As long as a laboratory moves at uniform speed and in a straight line relative to a laboratory at rest, it is impossible to discover in it any differences in the behavior of objects compared to the laboratory at rest. But as soon as the speed of the moving laboratory changes in magnitude (acceleration or deceleration) or in direction (curve), this immediately shows up in the behavior of objects in it.
Rest Is Lost For Good
The uniform straight-line motion of a laboratory has a surprising property. It does not influence the behavior of objects within it. This forces us to reexamine the notion of rest. It turns out that the state of rest and the state of uniform straight-line motion do not differ from each other in any way. A laboratory moving uniformly in a straight line relative to a laboratory at rest can itself be taken to be a laboratory at rest. This means that there exist not one absolute state of rest but an innumerable multitude of different "rests." There exist not one laboratory "at rest" but an innumerable multitude of laboratories "at rest," all moving in straight lines and uniformly at different speeds relative to one another.
Since rest has proved to be not absolute but relative, we see that motion also must be relative in all cases.
We must always answer the question, "Relative to which state of 'rest' is the motion being observed?"
So we have arrived at a most important law of nature, which is usually called the principle of the relativity of motion. It says: In all laboratories moving in straight lines and uniformly with respect to each other the motion of objects takes place according to the same laws.
The Law of Inertia
It follows from the principle of relativity of motion that an object on which no external force acts can be not only in a state of rest but also in a state of uniform straight-line motion. This statement is known is physics as the law of inertia.
However, in everyday life this law is, so to speak, obscured and not directly in evidence. According to it, an object in a state of uniform straight-line motion should continue this motion indefinitely, unless external forces act on it. But we know from observation that objects to which we do not apply forces tend to come to rest.
The explanation is that all objects that we are able to observe are subject to certain external forces—the forces of friction. The condition required for the law of inertia to hold good does not exist—namely, the total absence of external forces acting on the body. But if we keep improving the conditions of the experiment and reducing the frictional forces, we can get closer and closer to the ideal condition necessary. In this way we can prove that this law is also valid for motions observed in everyday life.
(Continues...)
Excerpted from What is Relativity? by L. D. Landau, G. B. Rumer, N. Kemmer. Copyright © 2003 Dover Publications, Inc.. Excerpted by permission of Dover Publications, Inc..
All rights reserved. No part of this excerpt may be reproduced or reprinted without permission in writing from the publisher.
Excerpts are provided by Dial-A-Book Inc. solely for the personal use of visitors to this web site.
Table of Contents
1 FAMILIAR EXAMPLES OF RELATIVITY2 SPACE IS RELATIVE
3 THE TRAGEDY OF LIGHT
4 TIME PROVES TO BE RELATIVE
5 CLOCKS AND RULERS PLAY TRICKS
6 WORK CHANGES MASS
SUMMING UP
INDEX